Antimicrobial surfaces
By Linda Geddes for Imperial Enterprise
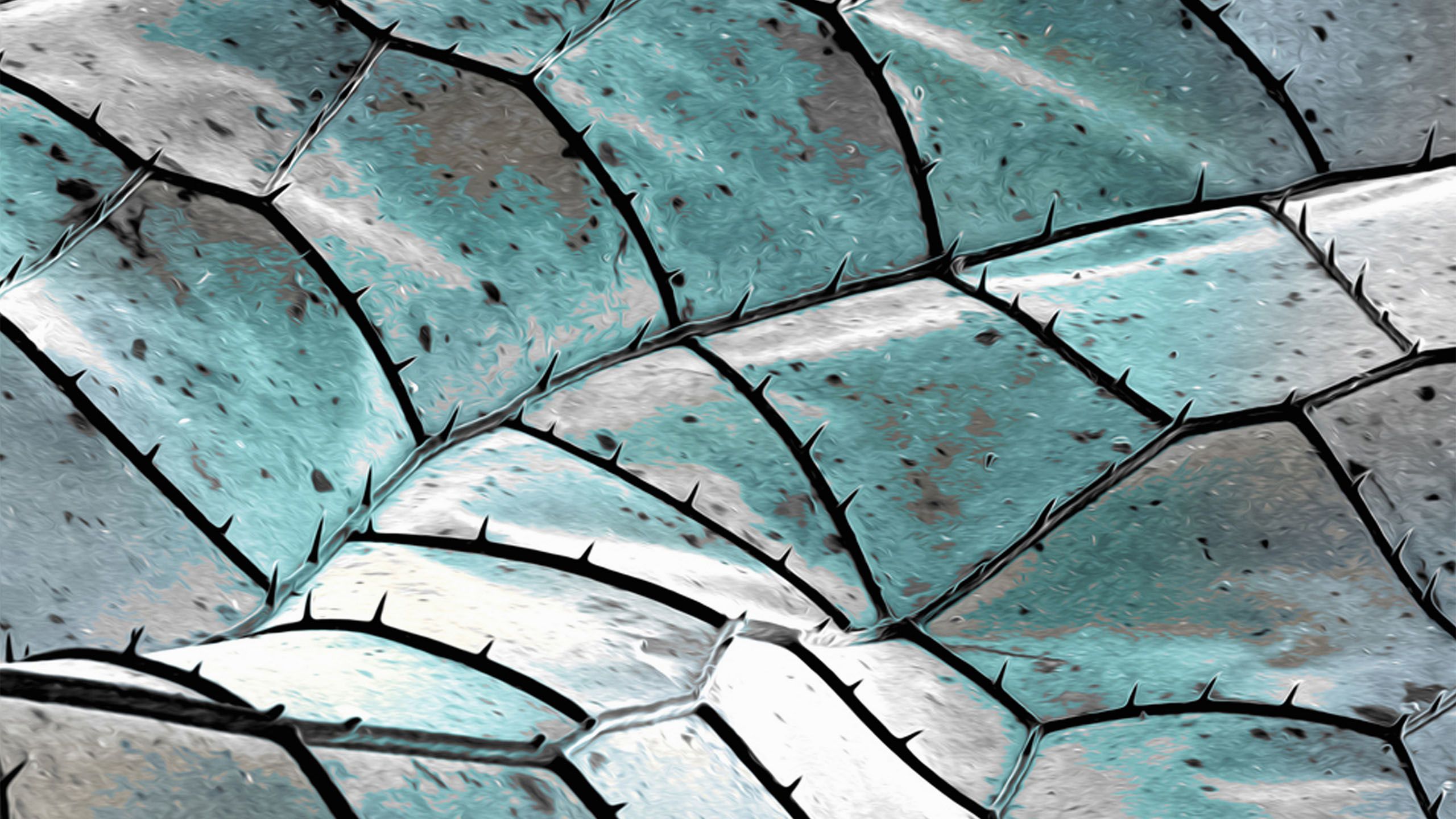

The survival of microbes on surfaces not only contributes to illness but to the emergence and spread of antimicrobial resistance.
The survival of microbes on surfaces not only contributes to illness but to the emergence and spread of antimicrobial resistance.
The coronavirus pandemic has thrown the role which surfaces play in the spread of disease into the spotlight – but viruses aren’t the only pathogen to be transmitted in this way. Disease-causing bacteria, fungi and fungal spores can also survive for long periods outside the body on objects and surfaces in our homes, hospitals and public spaces.
It started with a contaminated telephone handle. Within four hours, viral DNA could be detected throughout the intensive care unit (ICU) pod - on computer mice, door handles, ventilator knobs and medical charts. In the following days, it spread to five neighbouring pods, each housing four to eight vulnerable infants, and to the staff changing areas and break room.
Fortunately, no-one got sick this time. The viral DNA came from the cauliflower mosaic virus, which infects cauliflowers and related plants, but not humans - and it was deliberately wiped onto the telephone by researchers studying how microbes are transmitted through hospitals. Even so, the experiment demonstrated an important point: “It illustrates the challenges of the inanimate environment in health care and showed how important surfaces are in the transmission of bacteria and other microbes,” says Dr Jon Otter, an epidemiologist focused on Infection Prevention and Control at Imperial College London.
The coronavirus pandemic has thrown the role that surfaces play in the spread of disease into the spotlight – but viruses aren’t the only pathogen to be transmitted in this way. Disease-causing bacteria, fungi and fungal spores can also survive for long periods outside the body on objects and surfaces in our homes, hospitals and public spaces; when we touch these surfaces, and subsequently go on to touch our faces or other people, we can transmit infections.
It is a particular issue within hospitals, where the survival of microbes on surfaces not only contributes to illness but to the emergence and spread of antimicrobial resistance. A 2016 Public Health England point prevalence survey of more than 48,000 patients found that some 6.6 percent of patients acquired an infection as a result of their hospital stay. “If you are admitted to a hospital room where the previous occupant had certain types of micro-organism, say MRSA or [antibiotic-resistant] C. difficile, your risk of acquiring that micro-organism is doubled or more,” says Dr Otter. “Your hospital room can make you sick.”
Indeed, for vulnerable patients - such as those on intensive care wards; undergoing organ transplants; or receiving treatment for burns or cancer - acquiring one of these infections could spell the difference between life and death. “If we can improve our ability to remove contamination, we should mitigate that increased risk and improve outcomes for patients,” Dr Otter says .
Because of this, researchers at Imperial’s Institute for Molecular Science and Engineering (IMSE) are investigating the potential role of ‘smart surfaces’ in the fight against disease, as well as developing and testing some themselves. These new materials are designed to make it harder for microbes to attach, and if they do become established, the surfaces are engineered to either kill them outright, or make it far easier to rinse them off.
“At the moment, we are focused on applying such surfaces in the clinic, but they could also be implemented in offices, public transport, even in the home,” says Dr Gerald Larrouy-Maumus, a senior lecturer in molecular microbiology at the MRC Centre for Molecular Bacteriology and Infection.
Such materials could be used to manufacture countertops, doors and bedrails, as well as surgical tools. They could be used to line the inside of medical devices such as urinary catheters or the external surface of artificial hips, where contamination with bacteria can lead to serious and difficult-to-treat infections. They could also be incorporated into water pipes or drains to reduce the growth of slimy build-ups of bacteria called biofilms. Once established, these biofilms are notoriously difficult to remove, because antibiotics and disinfectants can’t penetrate through the slimy glue that the bacteria secrete to protect themselves.
To make matters worse, these biofilms can contribute to the problem of antimicrobial resistance directly, because individual bacteria living in the colony can share and swap genetic material conferring antimicrobial resistance between them, helping it to spread. Drains, in particular, are emerging as an important source of antimicrobial resistant bacteria within hospitals, because they collect the bacteria which are washed off people’s hands and surfaces, providing a reservoir in which they can multiply and share information in this way .
Deadly surfaces
In March, IMSE launched a briefing paper assessing the current state-of-the art for such antimicrobial surfaces and summarising the various approaches that might be taken to manufacture them. One conclusion was that more research is urgently needed - yet already engineers, clinicians, microbiologists and infectious disease experts at Imperial are developing technologies which could provide part of the solution .
So, what might an antimicrobial surface look like? Various approaches are being explored, but probably the most advanced is using materials with innate antimicrobial properties - or bestowing such properties on materials like plastics through engineering.
Consider copper: People have been using this metal to prevent infections for millennia. Ancient Greek and Babylonian soldiers would place the bronze filings (bronze is an alloy of copper and tin) produced by sharpening their swords into their wounds to hasten healing, while in India, traditional Ayurvedic practitioners have long-instructed people to store their water in copper vessels to improve their health. Copper destroys bacteria by releasing electrically charged particles called ions when they land on its surface. These ions enter bacterial cells and interfere with essential processes such as energy production (respiration) and protein synthesis, as well as punching holes in their cell membranes.
The US Environment Protection Agency found that copper alloys kill 99.9% of disease-causing bacteria within two hours, while a 2013 study conducted in three US hospitals found that switching the materials of six high-contact surfaces in patients’ rooms – including bed rails, pull-down tables, visitor chair arms and nurse call buttons – from plastic to copper, resulted in a 58% reduction in healthcare-associated infections. “As a proof of concept, that’s pretty powerful,” says Dr Otter. Copper also destroys viruses – including coronaviruses - by disrupting their protein shell and damaging their genetic material.
Because of such findings, copper is increasingly being deployed within research labs, such as those at the Francis Crick Institute in London; in high-end kitchens; and being used to replace some high touch surfaces - those handled frequently throughout the day by numerous people, e.g. light switches, handrails and door handles - within theme parks, airports and train stations.
Yet copper isn’t perfect. For one thing, it’s relatively expensive. “If this approach [of deploying antimicrobial surfaces] is going to be implemented on a mass scale in clinical environments, it will need to be made more cost-effective,” says Dr Otter. “There are also some patient acceptability issues that we need to think through: after all, who wants to sit on a copper toilet seat?”
This isn’t the only problem with copper. Mycobacterium abscessus is a hospital bug which is associated with surgical infections and complications in patients with existing lung diseases, like cystic fibrosis. It is also the most common source of contamination within hospital water systems – and it is becoming increasingly difficult to treat because of growing resistance to several antibiotics. “M. abscessus is a nightmare for hospitals,” says Dr Larrouy-Maumus.
The bacterium likes to colonise water pipes, so Dr Larrouy-Maumus and his colleagues decided to investigate whether certain trace elements, like copper, which leach from these surfaces could promote or inhibit its growth. “We found that this bacterium adapts very well to copper – and depending on the drug, can even become more resistant to some antibiotics in its presence,” Dr Larrouy-Maumus says. Possibly, this is because copper triggers a bacterial defence mechanism, prompting the bug to alter its metabolism, gene expression, and the structure of its surface envelope. In so doing, the action of certain antibiotics is inadvertently altered.
The good news is that M. abscessus appears to be damaged by nickel ions; embedding some nickel into water pipes might therefore inhibit its growth – although the effect of this metal on other types of bacteria will need to be explored. “Our ultimate aim is to find a material which will inhibit all microbes,” Dr Larrouy-Maumus says.

At a border control point in Santiago Airport, Chile, a copper surface is used to help prevent infections being spread among the many passengers passing through.
At a border control point in Santiago Airport, Chile, a copper surface is used to help prevent infections being spread among the many passengers passing through.
Copper destroys bacteria by releasing electrically charged particles called ions when they land on its surface. These ions enter bacterial cells and interfere with essential processes such as energy production (respiration) and protein synthesis, as well as punching holes in their cell membranes.
How could smart surfaces help tackle infection and antimicrobial resistance? - video by the Institute for Molecular Science and Engineering
How could smart surfaces help tackle infection and antimicrobial resistance? - video by the Institute for Molecular Science and Engineering

Hospitals aren’t the only environment in which antimicrobial materials can be deployed. Professor Williams is devising ways of reducing the growth of biofilms within the pipes of their manufacturing plants, which make various gels and liquids
Antimicrobial plastics
Of course, not all surfaces are metal; neither can they necessarily be replaced by metal. So, Daryl Williams, a professor of particle science at Imperial’s department of chemical engineering, and his colleagues are exploring the possibility of applying metal nano-coatings to plastics and other synthetic polymers which would make them less attractive, and more deadly to microbes. “There are lots of materials that are made from polymers - both disposable things used in medicine, but also general items within the home,” says Professor Williams. “We're currently focusing on developing coatings for polypropylene and polyethylene which gives them good antimicrobial properties they didn’t have before. We are currently discussing a study to assess their COVID-19 performance."
Other groups outside Imperial are also taking this approach. For instance, urinary catheters coated in a silver-impregnated gel are currently being tested which may reduce the incidence of hospital-acquired urinary tract infections.
However, hospitals aren’t the only environment in which Professor Williams sees such materials being deployed. His group has received funding to devise ways of reducing the growth of biofilms within the pipes of their manufacturing plants, which make various gels and liquids, including shampoo, skin care, and household cleaning products. “With any liquid system you are going to get biofilms forming at some point, and if they decide to detach themselves that could affect your final products,” Professor Williams says.
Inspired by nature
Engineering surfaces which are toxic to bacteria is just one of the approaches being explored by IMSE scientists. Another is to alter the surface topography of materials to make them less hospitable to microorganisms. For instance, the wings of cicadas and dragonflies are studded with tiny spikes, which though imperceptible to human eyes, are deadly to many bacteria - including Escherichia coli, Pseudomonas aeruginosa, Bacillus subtilis and Staphylococcus aureus, which are responsible for various hospital-acquired infections. The nano-needles puncture their cell walls, causing their contents to spill out, as well as triggering further internal damage through the release of free radicals. Even if they don’t manage to puncture the bacteria, they can also interfere with communication between individuals within a biofilm.
Researchers, including Jason Riley, a professor of materials electrochemistry, are drawing inspiration from these natural surfaces to develop antimicrobial surfaces of their own. “One surface won’t destroy all bacteria, but you could develop a surface to deal with certain types of bacteria,” he says. For instance, his lab has been experimenting with layering various spiky particles onto surfaces, and their impact on bacterial growth is now being investigated. A lot of it has to do with surface charge, he explains. Many bacteria carry a negative surface charge, so if the spikes on a material’s surface carry a positive charge, the bacteria will be brought towards them through electrostatic attraction, and impaled. “If I change the surface charge, I can change which bacteria I attract,” Professor Riley says.
One class of molecules being investigated by various labs for controlling surface charge is the organosilanes. Silicon-carbon based nanocoatings can be attached to both soft and hard materials, meaning they could be deployed on many different types of surfaces, including bedrails, clothing, carpets, or plastic tubes. They could also be retrofitted to existing surfaces and equipment within hospitals, rather than having to rip the old ones out or buy replacements, thereby reducing costs. However, although some studies have suggested that organosilane coatings can significantly reduce the number of bacteria on surfaces, others have shown no effect. Possibly, this is because of differences in the composition of bacterial cell walls, rendering some species more susceptible to being pierced by nanospikes than others.
“I’m pretty convinced that we are going to have to take various methods and marry them together to get the most effective surface,” Professor Riley says. For instance, it may be possible to combine nanospikes which his team is developing with copper, or with some of the antimicrobial coatings being developed in Professor Williams’ lab.

The wing of a dragonfly, photographed with an electron microscope. Photo: Cultura/Gregory S. Paulson via Getty Images
The wing of a dragonfly, photographed with an electron microscope. Photo: Cultura/Gregory S. Paulson via Getty Images
The wings of cicadas and dragonflies are studded with tiny spikes, which though imperceptible to human eyes, are deadly to many bacteria

Computer illustration of staphylococci aureus bacteria, a common cause of hospital-acquired infections. The bacteria can infect almost any site in the body and are often resistant to antibiotics. Image: Kateryna Kon/Science Photo Library via Getty Images
Computer illustration of staphylococci aureus bacteria, a common cause of hospital-acquired infections. The bacteria can infect almost any site in the body and are often resistant to antibiotics. Image: Kateryna Kon/Science Photo Library via Getty Images

3D illustration of a material inspired by shark skin. Image: xrender via Getty Images
3D illustration of a material inspired by shark skin. Image: xrender via Getty Images
Shark skin
Other nano- or micro-scale physical features might also be in incorporated, such scales or ridges. An example of this is Sharklet™, a surface which also draws inspiration from nature – in this case, shark shin. Sharklet was developed by researchers at the University of Florida, initially as a means of keeping the hulls of ships and submarines free of algae, another type of biofilm. Seeking examples of slow-moving marine creatures which don’t become coated in slime, they alighted on sharks, whose skin possesses tiny scale-like projections called denticles, arranged into ridges in a distinct diamond pattern. This topography exerts mechanical stress on any bacteria which settle on it, disrupting their normal function and forcing them to expend more energy just to survive, meaning they must peel off or die.
This micropattern, has since been reproduced in on the surface of various materials, including acrylic and silicone. One promising application is in reducing the migration of bacteria such as E. coli up catheter tubes – a major source of urinary tract infections. Sharklet-patterned films have also been developed which can be applied to flat surfaces, such as doors and countertops. When applied to such high touch surfaces, Sharklet reduced contamination with antimicrobial resistant Staphylococcus aureus (MRSA) by as much as 94 percent.
Self-cleaning
Such surface modifications could also be combined with self-polishing coatings, similar to the anti-fouling paints which are applied to the hulls of ships. Many of these rely on the use of sea water-soluble pigments such as copper oxides, which are toxic to many bacteria, and are constantly sloughed off through the action of water flowing over them. A similar concept might be applied to healthcare settings - e.g. in water pipes or drains, where there’s an existing flow of liquid to remove the outer coating - provided a suitable antimicrobial agent can be identified, and which isn’t harmful to people’s health or the environment.
Photocatalytic coatings are another possibility. These can be painted onto surfaces, and release free radicals - which attack bacterial cell membranes, and viral proteins and genetic material - upon exposure to light. For instance, titanium dioxide has been successfully deployed on hospital tiles and windows, as well as on silicone catheters, which can be sterilised by irradiating them with ultraviolet light.
“What we are shooting for is the emergence of some kind of platform technology, which would cover all of the application areas – hospital surfaces, surgical tools and medical devices, and hospital water systems - and which merges these various modes of action,” says Dr Otter.
Antimicrobial surfaces clearly have the potential to reduce microbial attachment, kill disease-causing organisms and make hospitals easier to clean. This approach is also very different to the measures currently used to reduce the transmission of infections, such as disinfectants and antibiotics. Indeed, the overuse of antibiotics, both in healthcare and agriculture, has significantly contributed to the development and spread of antibiotic-resistant bacteria - and tackling it is high on the agenda for many governments and scientists. In Europe and the US alone, there are around 50,000 deaths resulting from antimicrobial resistant infections, each year, and this is predicted to increase unless new solutions can be found.
Antibacterial surfaces could reduce some of our reliance on antibiotics. Also, because such surfaces tend to either physically destroy the bacteria (e.g. by puncturing them), or destroy their DNA, the bacteria are far less likely to develop resistance to them.
Yet designing, manufacturing and testing these new technologies will require close collaboration between scientists from many different disciplines: “To design these surfaces we will need surface engineers – people who can fabricate them to the right specifications – as well as medics, and people with expertise in infectious diseases to test them and ensure that they are actually killing bacteria,” says Kieran Brophy, communications manager at IMSE. Finding ways to manufacture these surfaces cheaply will provide an additional challenge, and since the introduction of new surfaces will undoubtedly incur significant costs healthcare economists must be involved in these discussions from the outset, to ensure they represent good value for money.
“We want to develop smart surfaces that are affordable, and not restricted to hospitals or clinics which can afford them,” says Dr Larrouy-Maumus. This includes low- and middle-income countries, where they could help overcome additional obstacles to infection control, such as reduced access to clean water and electricity.

Antibacterial surfaces could reduce some of our reliance on antibiotics. Also, because such surfaces tend to either physically destroy the bacteria (e.g. by puncturing them), or destroy their DNA, the bacteria are far less likely to develop resistance to them.
Other antimicrobial innovations
Imperial professors Mimi Hii and Klaus Hellgardt have developed a novel device that can be fitted to home-care appliances to generate hydrogen peroxide or bleach, effectively producing a ‘self-cleaning’ appliance. Their invention is an electrochemical system capable of ‘smart’ dispensing of inorganic oxidants locally, which can be used for disinfection purposes, e.g. toilet cisterns, sterilisation, removal of odours. Their technology is currently available for licensing.
Dr Chiara Hyde founded her startup BrightCure as a PhD student in Imperial's Department of Chemical Engineering. The company is developing a technology to treat urinary tract infections without antibiotics using localised light therapy aimed at killing bacteria in the bladder.
Even without virus-specific surfaces, the development of antibacterial surfaces could help to reduce to the death rate from COVID-19 and other viral infections such as influenza.
A combined approach
Promising as antimicrobial surfaces may be, the spread of infection and antimicrobial resistance will not be tackled by antimicrobial surfaces alone. “If we absolutely nail surfaces and do nothing else, it will be futile,” says Dr Otter. “They are not a silver bullet.” Instead, they should form part of a package of measures to reduce disease transmission, alongside responsible antibiotic use and more basic infection control measures, such as regular handwashing.
Also, whereas the current focus is on antibacterial surfaces, there is a clear need to develop surfaces which could similarly impede the transmission of viruses and disease-causing fungi. Copper and titanium dioxide are two examples of surfaces or coatings which kill all these organisms, but there will surely be others. And although nanospikes are too large to be able to puncture viruses, these could be engineered to trap viral particles and prevent them from being transferred to other surfaces such as people’s hands. Disinfectants could then be applied to destroy these trapped viruses, Dr Larrouy-Maumus suggests.
Yet, even without virus-specific surfaces, the development of antibacterial surfaces could help to reduce to the death rate from COVID-19 and other viral infections such as influenza. Dr Larrouy-Maumus has applied for funding to study whether the deployment of antibacterial surfaces could reduce the rate of complications, such as bacterial pneumonia, in patients hospitalised with viral infections. One study of patients who were admitted to the ICU with COVID-19 in China, found that around half of those who died, did so because of a secondary bacterial infection, rather than the virus itself. “When people are in the ICU with coronavirus, they don’t stay for one or two days, they often stay for several weeks, and this drastically increases their chances of contracting a hospital-acquired bacterial infection, such as one that attacks the surfaces of the lungs,” says Dr Larrouy-Maumus.
To reduce this risk, ICU patients are often given antibiotics as a preventative treatment - but doing so may accelerate the development of antibiotic resistance within hospitals, making these bacteria harder to kill in the future.
The need for good infection control and reduced transmission of disease has never felt so pressing. With world-leading expertise in infectious disease, medical devices, the physics, chemistry and engineering of nanomaterials, and the generation of innovative and sustainable business solutions, Imperial is well placed to meet this challenge.
Further reading
- Download the IMSE briefing paper on antimicrobial surfaces and watch the video from the paper's launch event on the IMSE website.
This feature was produced by Imperial's Enterprise Division.
The Enterprise team helps businesses to solve their challenges by accessing Imperial's expertise, talent and resources, and helps staff and students find new ways to turn their expertise into benefits for society.
