Mission to the ocean moons
Could Jupiter’s icy moons hold the conditions for life?
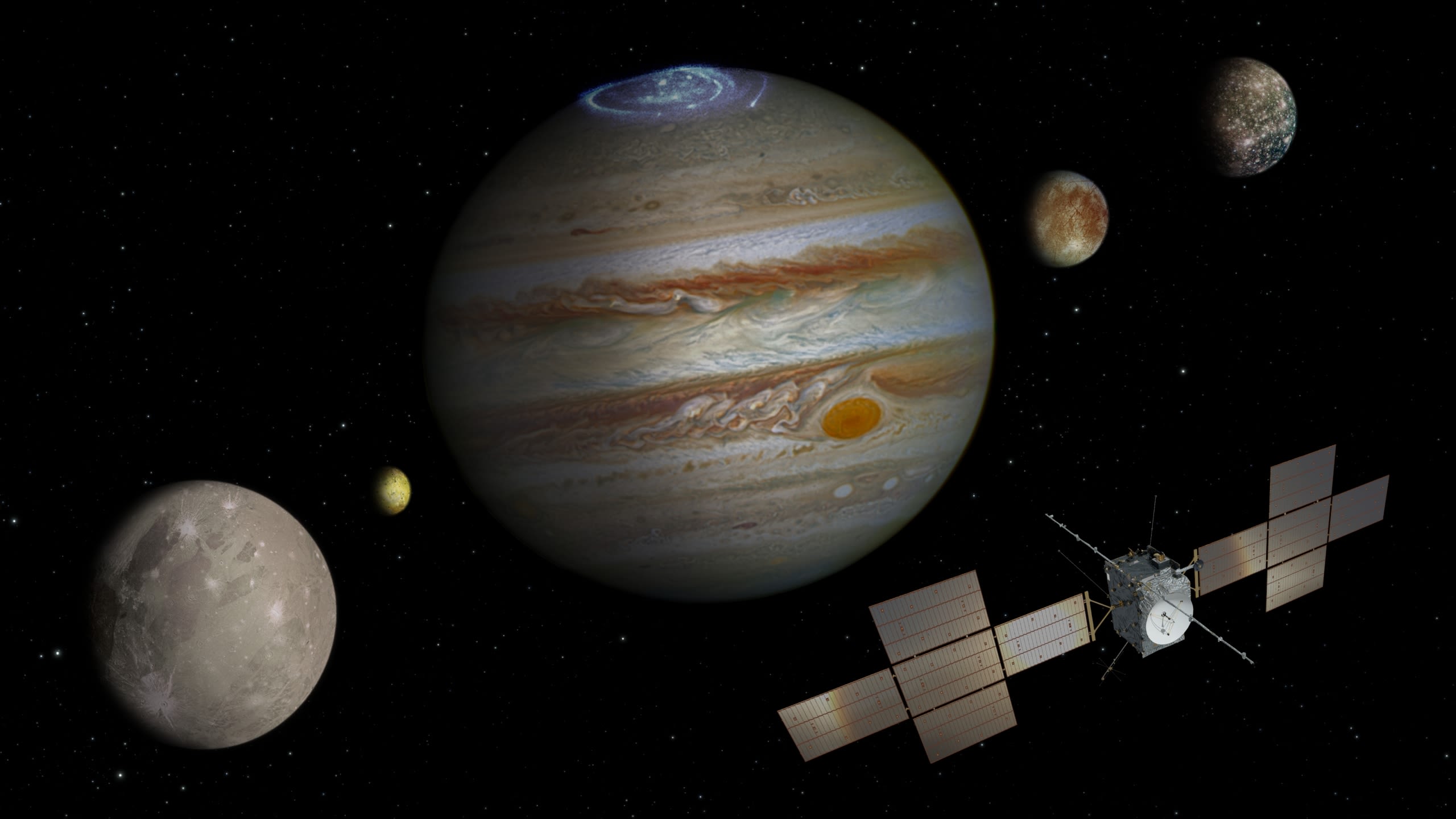
There are four conditions scientists think are needed for life to emerge: liquid water, a heat source, organic material, and for those three ingredients to be stable enough for long enough.
The need for liquid water has meant the search for life in our Solar System has concentrated on planets closer to the Sun. But we now know that the Sun’s warmth isn’t the only way to create liquid water.
Beneath the icy crusts of moons orbiting Jupiter, there are liquid oceans. As the largest moons orbit Jupiter and pass each other, they stretch and compress via the effects of gravity, causing them to heat up and some of the ice to melt.
Now, the European Space Agency’s Jupiter Icy Moons Explorer (JUICE) spacecraft is going to visit three of these moons, with a mission to find out if their oceans have the conditions for life.
On board is a piece of Imperial-built kit that is crucial to this mission.
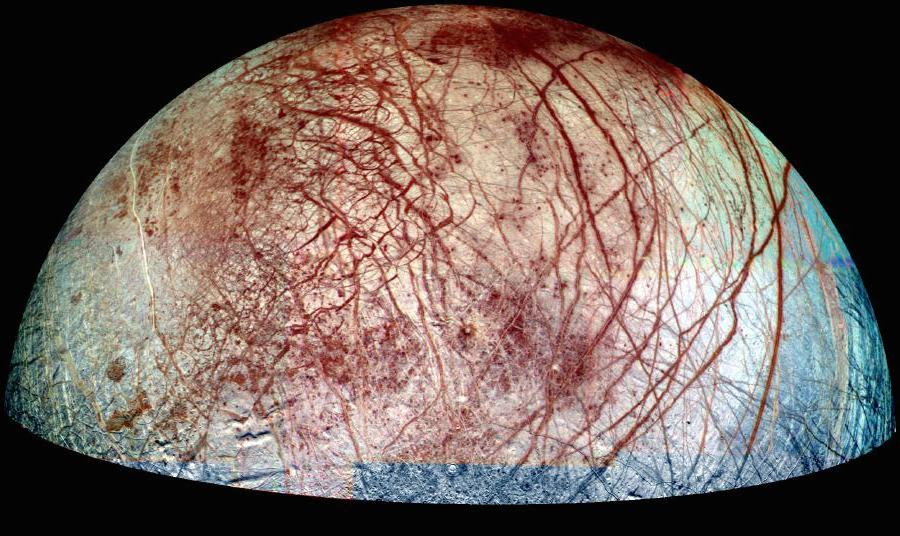
Exploring the moons
JUICE will visit three of Jupiter’s largest moons: Callisto, Europa, and Ganymede. It will fly by the first two multiple times, but at Ganymede it will do something never done before: it will go into orbit around a moon other than our own.
The moons are all expected to be ocean worlds, but are thought to have different characteristics. Europa, for example, is thought to have plumes of water steaming into space from cracks in the surface ice, much like Saturn’s moon Enceladus (a discovery only made possible by the Cassini spacecraft’s magnetometer instrument, also built at Imperial). Callisto’s structure is less certain and may throw up surprises.
Ganymede, however, is unique for several reasons. It’s the largest moon in the Solar System, larger than Mercury. It’s the only moon known to have its own magnetic field, suggesting a molten metal component to its core. And its liquid ocean is predicted to contain vastly more water than all Earth’s oceans put together – although it’s likely to be buried beneath kilometres of ice.

Professor Michele Dougherty, Head of the Department of Physics at Imperial, is the Principal Investigator for the magnetometer instrument aboard JUICE, named J-MAG. People often laugh when she says it’s the most important instrument on the spacecraft, but she’s not joking.
While all the instruments – including particle detectors, cameras, radar instruments and radiation monitors – will produce reams of useful data, the magnetometer data is crucial if scientists are to resolve some of the key questions about the moons’ oceans, including their depth and salt content, and whether the oceans are global or concentrated in certain areas.
Magnetometers measure the characteristics of magnetic fields, including their magnitude and direction. Magnetic fields can be created in a few ways, including via internal ‘dynamos’ – moving molten metals inside planets like Earth.
The oceans of the moons JUICE will visit are expected to be salty, such that the movement of currents within them leads to small magnetic fields. But these fields are embedded in larger fields: from the interior of Ganymede, from Jupiter, and from the Sun itself. All these need to be untangled to sense the oceans.
It’s this dilemma that keeps Professor Dougherty awake at night. “What we’re doing is extremely difficult,” she says. “It’s like trying to find lots of needles in a haystack, and those needles are changing shape and colour all the time.”

Measuring the magnetic field
J-MAG, however, is up to the challenge. It can sense magnetic fields five million times smaller than the Earth’s and is built to withstand the extreme temperatures and harsh radiation environment around Jupiter.
J-MAG consists of three sensors – one built at Imperial, one built at the Technical University of Braunschweig, Germany, and one built at the Space Research Institute, Graz in partnership with Technical University Graz, Austria – plus an electronics box and metres of cable assembled at Imperial.
The sensors will be placed along a 10.6-meter boom – twice as long as any ESA has ever built – to keep them away from any magnetic fields created by instruments on the main body of the spacecraft.

Test of the boom unfoldng, using balloons to simulate the lack of gravity
Test of the boom unfoldng, using balloons to simulate the lack of gravity
Imperial’s ‘outboard’ sensor, sitting near the end of the boom, is a fluxgate magnetometer. It's made up of a circular magnetic core (grey, below) in two halves. The core is made up of a material sensitive to magnetism, where many of its atoms act like tiny magnets, called dipoles.
A wire coil (blue) wraps around the core, capable of inducing a magnetic field. Finally, there is a rectangular wire wrap (orange) that senses the action of the dipoles.

It works like this (Jupiter is used as an example of the target):
This sensor works together with the other two to characterise a target magnetic field.
The complete magnetometer
Imperial's 'outboard' fluxgate sensor.
The German sensor, known as the ‘inboard’ sensor, sits three metres away from the tip of the boom. It is also a fluxgate design but uses smaller cores than Imperial's outboard sensor. By operating both inboard and outboard sensors together, the team can use them to detect and remove any residual magnetic fields caused by the spacecraft that would contaminate the science signal.
The Austrian sensor is a type never used beyond Earth orbit before, which uses quantum technology to measure the absolute magnitude of the magnetic field. This measure is essential for calibrating the other sensors, so they can get accurate data on all the characteristics of the magnetic field.
In previous space missions, this calibration has been performed by ‘rolling’ the spacecraft – turning it over as it travels a short distance and comparing the fields measured across two fluxgate sensors. But the magnetic environment around Ganymede is so complicated and changing so rapidly that rolling would not be useful, so another calibration method is needed.
The electronics box receives and processes the data.
All these complications mean the instrument has been challenging to design and build. But it was made even more challenging by the pandemic: with a strict schedule to keep to, in 2020 the Imperial team originally took home parts to tweak on their kitchen tables.
Instrument Manager for J-MAG Patrick Brown said: “We were pulled from going to go to a test when College told us that we were shutting down. But they let us back in only four weeks after the shutdown, and though only a few of us were here at once, we were able to get the instrument built and delivered on time.”
During the mission, the team are also partnering with the University of Leicester, who are responsible for radiation analysis, and Kyoto University, Japan, who will support the in-flight calibration of J-MAG.
J-MAG vital statistics:

Instrument Engineer Richard Baughen (left) and Instrument Software Engineer Alex Strickland (right) work on one of the instrument models
Instrument Engineer Richard Baughen (left) and Instrument Software Engineer Alex Strickland (right) work on one of the instrument models

Senior Instrument Manager Patrick Brown
Senior Instrument Manager Patrick Brown
A long way to go
JUICE will launch atop an Arianne 5 rocket, the most powerful at ESA’s disposal, from their spaceport in Kourou, French Guyana. Even with all that power, however, it’s not enough to get to Jupiter directly – the spacecraft will use the Earth and Venus for a few gravity assists to swing it out towards Jupiter.
JUICE is expected to arrive in the Jupiter system in 2031, flying by the planet and the moons Europa and Callisto before going into orbit around Ganymede in 2034. The magnetometer will switch on every six months on the way to check its performance, and may even squeeze in a little science on the way, measuring the way the Earth’s magnetic field changes during the flybys and comparing data with other craft out in the Solar System.
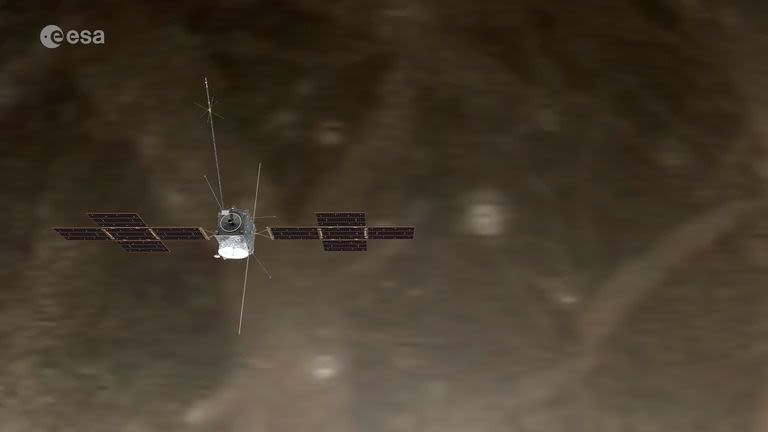

Dr Shivangi Sharan
Dr Shivangi Sharan
This will keep the engineering team busy, but the scientists won’t be idle while the instrument is dormant either. Dr Shivangi Sharan, a new addition to the team, has come on board to help figure out what data might be the most useful.
Our present knowledge about Ganymede comes mostly from the Galileo mission, which flew by taking measurements from 1996-2003, and from remote observation by the Hubble Space Telescope. These data are useful for creating different models that best describe the field measured. Researchers like Dr Sharan can then predict what kind of data JUICE should see based on those different models, so that if we find data that aligns well with one of them, we already have a good understanding of what this means.
Even with this preparation, the answers won’t come right away. The end of the mission is predicted to be 2035, although if there’s enough fuel it may last a little longer (and get a little closer to Ganymede), and the data will take a while to analyse.
Professor Dougherty says: “Space missions are long and slow, so launch only marks the halfway point of this one – because we first started thinking about it 15 years ago, and we'll be getting the last data in 15 years’ time. But I can't wait for launch to happen because that's the next milestone for us – we’ll be on our way to Jupiter.”

The Imperial J-MAG team. L-R: Dr Adam Masters, Patrick Brown, Dr Shivangi Sharan, Alex Strickland, Professor Michele Dougherty and Richard Baughen
The Imperial J-MAG team. L-R: Dr Adam Masters, Patrick Brown, Dr Shivangi Sharan, Alex Strickland, Professor Michele Dougherty and Richard Baughen
Illustrations and animations of JUICE all credit to ESA/ATG medialab. Europa image courtesy of NASA.Photos of Imperial's JUICE team and instrument credit to Thomas Angus, Imperial College London. Illustrations of magnetometer credit to Imperial College London/Richard Palmer.