Chemistry built the modern world. Can it save it too?
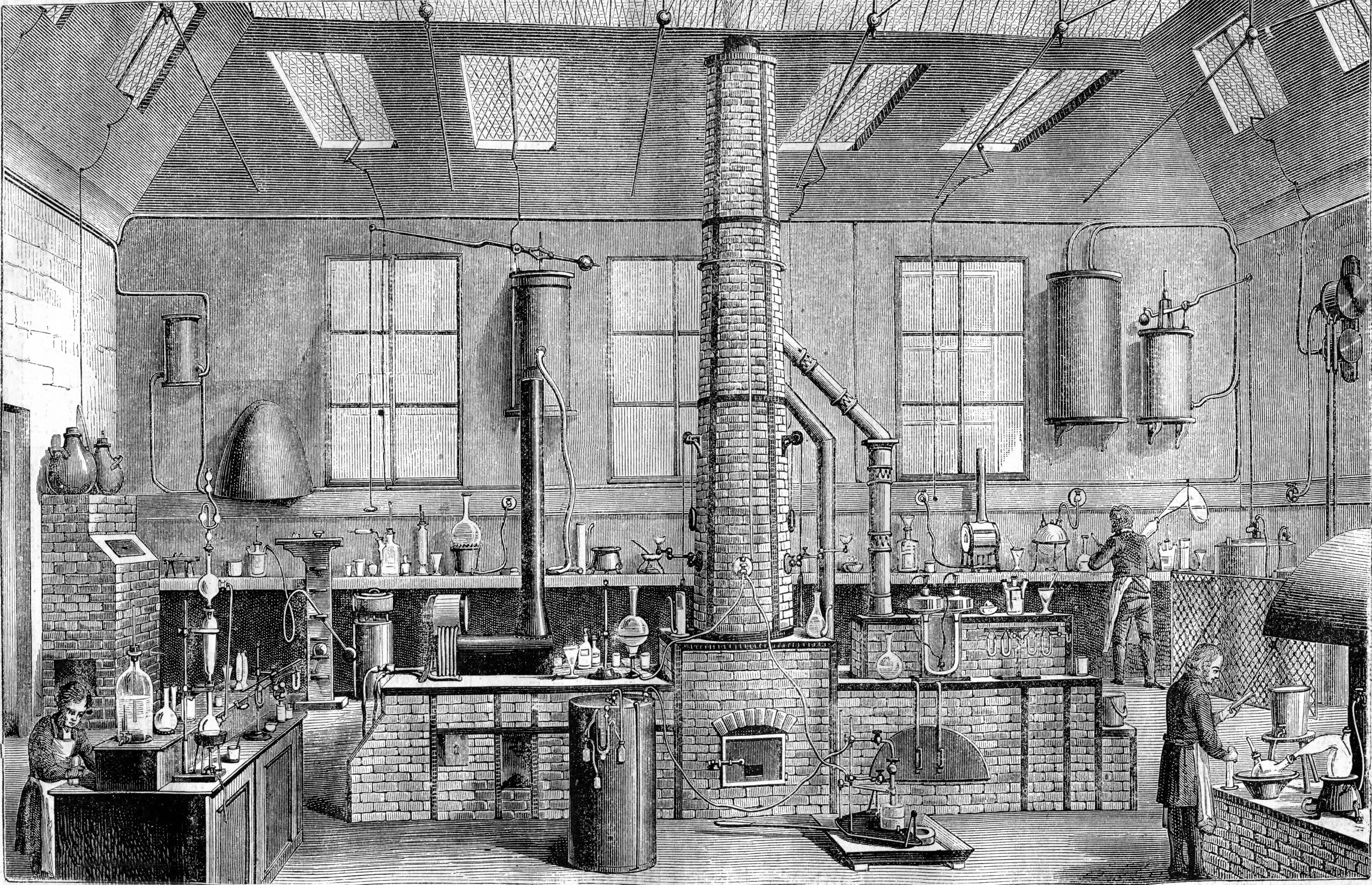
Building a more sustainable future for our people and our planet will require a step change in the way we make chemicals and materials. That is the focus of Imperial’s new Institute for Digital Molecular Design and Fabrication (DigiFAB).
Chemistry has arguably played a greater role in the twists and turns of civilisation than any of the other sciences. Our ancient ancestors learned to smelt different metals together to make new, harder alloys, such as bronze, that yielded better properties than their constituent parts, paving the way for more advanced tools and weaponry.

Impression of a bronze age foundry
Impression of a bronze age foundry
Later, we discovered how to make concrete, cement and steel to build great cities and eventually learned how to extract and refine fossils fuels to power this on-going expansion.
This process of discovering and creating new molecules and materials with desirable properties has built the modern world as we know it – for better or worse – and continues today.

Now our biggest challenge lies in securing a more sustainable future for our people and our planet. This will entail, for example, developing new molecules and materials that can capture carbon dioxide; solar cells that can more efficiently convert sunlight into energy; and advanced materials that can store this energy stably in batteries. We also need to protect ourselves from disease and illness and improve the quality of life for people around the world.

An oil refinery and petrochemical plant
An oil refinery and petrochemical plant
Arguably we’re at a unique juncture in civilisation where these technological challenges are pressingly urgent and perhaps even contingent on our very survival in the case of the climate emergency. The coronavirus pandemic has also shown us that our health and way of life could be threatened by emerging infections – while the spectre of antibiotic resistance remains a major concern.
While our scientists continue to make incremental advances in these areas, we need to do more and at greater speed and scale.

Flexible solar cells made from ruthenium
Flexible solar cells made from ruthenium
That calls for a radical step-change in the way molecular science is done. It means utilising the power of data science, artificial intelligence (AI) and automation. More than that though, it requires a shift in thinking, where we select the material properties we need, driven by the major global challenges, and then effectively design them from the ground up.

Photo by Markus Spiske on Unsplash
That is the focus of Imperial’s new Institute for Digital Molecular Design and Fabrication (DigiFAB), which aims to launch a new era of digital chemistry. It is a multi-disciplinary institute led by Professor Sophia Yaliraki, Professor of Theoretical Chemistry in Imperial’s Department of Chemistry.

Professor Sophia Yaliraki, Professor of Theoretical Chemistry and Director of the DigiFAB Institute
Professor Sophia Yaliraki, Professor of Theoretical Chemistry and Director of the DigiFAB Institute
She comments: “If you look at the history of chemistry and chemical discovery, up until relatively recently, it hasn't always been quantitative – if you think about how it started with people tasting and smelling from flasks and bottles. In the 20th century it became more rigorous, but really just for very small molecules and limited accuracy. If you’re trying to solve big problems at scale you need to think what the chemical and molecule is doing in its wider surroundings – you need a very new set of rules.”

The Molecular Sciences Research Hub (MSRH), where DigiFAB is based.
The Molecular Sciences Research Hub (MSRH), where DigiFAB is based.

The current state of play

At present, chemical and material discovery programmes tend to be relatively conservative, typically involving researchers making small incremental chemical modifications to known materials. In some cases, materials are re-examined and screened for new applications and trial and error and serendipity also play a role, as they have always done in science.
This approach has served us reasonably well up to now – but it is slow, costly, inefficient and tends to be energy intensive. It’s often said that for a drug to go from discovery to market, it usually takes more than a decade and $1 billion. As a result, there hasn’t been a new class of antibiotics brought to market for decades, despite rising incidences of drug-resistant infection. Those timescales and costs can be even higher for agrochemicals and advanced materials, with some estimates suggesting it can take up to 40 years from laboratory discovery to industrialisation.
Partly because of this protracted timescale, chemists can be unwilling to take large risks and tend to focus on synthesising compounds where they can be confident of success, often at the expense of large leaps forward in property performance.
This also means that we have only explored the tiniest fraction of potential ‘molecular space’ out there. The chemical space of drug-like organic molecules alone, which are also potential material building blocks, is estimated to be between 1023 and 1060 possible compounds. Indeed, looking at organic and inorganic compounds, there are more ways to combine molecules than there are atoms in the solar system.
That is both a tremendous opportunity and a tremendous challenge – and one that requires the power of digital techniques to truly unlock.
We are seeking to redefine the entire landscape of chemistry. And by that, we just don't mean focusing on the molecule itself...
And it’s worth remembering that materials can be simple systems made from single molecules or a small number of elements, or they can be more complex systems processed into a variety of forms, including crystals, amorphous structures, thin films, and devices assembled from multiple materials.

Professor King Kuok (Mimi) Hii, DigiFAB co-lead for the Synthesis and Processes, Department of Chemistry
Professor King Kuok (Mimi) Hii, DigiFAB co-lead for the Synthesis and Processes, Department of Chemistry
Professor Mimi Hii, DigiFAB co-lead for the Synthesis and Processes research pillar comments:
“We are seeking to redefine the entire landscape of chemistry. And by that, we just don't mean focusing on the molecule itself, of course that's very important, but actually across the entire length scale – from tiny little electrons and atoms right through to processing. So while we discover things in the lab, at the same time we’re thinking about how to scale that up to manufacture – be it a drug, agricultural product or a new material – so that society can benefit.”



The holy grail of inverse design

In recent years chemists have been collecting, and crucially sharing, an increasing body of data on precursor molecules which can form the basis of new compounds, materials and devices. These databases contain crucial information about the chemical properties and behaviour of precursors, often at the quantum level.
Dr Felice Torrisi, DigiFAB’s co-lead for Sensors and Characterisation comments: “In the past, chemists used to handle data individually for every experiment. You have your data, you analyse that and that becomes your single package and then you move to another experiment.
In the past, chemists used to handle data individually for every experiment. You have your data, you analyse that and that becomes your single package and then you move to another experiment.
“We’re shifting to a more holistic approach of handling the data globally, which requires a proper established way to process, manage and analyse. This is also extremely important in terms of translating scientific research into innovation in industry.”
With these global databases in place, researchers can start to perform data-mining approaches – for example extrapolating the properties of a compound or material from combinations of precursors that have already been explored. In this way, you can start to make inferences about completely new classes of materials. Introducing artificial intelligence (AI) and machine learning (ML) into this picture opens up even greater possibilities.
You can train AI/ML on known combinations, the more of which you can expose it to, the more it learns, until it can start to make suggestions and run simulations for new compounds and materials. Sometimes AI can even make ‘wild card suggestions’ that would simply not have occurred to a human operator – according to Dr Kim Jelfs DigiFAB co-lead for Data and Modelling.

Photo by Markus Spiske on Unsplash
Perhaps the most high-profile recent example of the use of AI in the molecular space (although not for discovery per se) is from Google’s Deep Mind. The company uses its proprietary algorithms to predict the complex and difficult-to-predict folded structure of proteins based on the sequence of amino acids that make them up. This normally requires painstaking, multistep crystallisation – meaning that after decades of research just 17% of human proteins had been mapped. But using this small subset to ‘train’ Deep Mind’s algorithms, the company has now predicted the structure of 98.5% of all proteins in just under a year – providing an immensely powerful resource for biologists and synthetic chemists.

3D structure of human pepsin protein. Elucidating the way these proteins fold is an immense challenge.
3D structure of human pepsin protein. Elucidating the way these proteins fold is an immense challenge.
The next step in inverse design is to use automation and robotics to actually synthesise the compounds suggested from the design phase, and also to test, analyse and characterise their molecular properties. This in turn generates yet more data for the design and discovery phase – effectively closing the loop of a virtuous circle which leads to the continuous refining, tweaking and improvement of materials and compounds.
This type of inverse design also aims to make chemical development more efficient, which will accelerate discovery and improve sustainability by reducing waste in the process.
Breaking down silos

DigiFAB is now putting in place the foundations to make this vision for digital chemistry a reality – in terms of its people, partners and facilities.
The completion of the Molecular Science Research Hub (MSRH) at Imperial’s White City Campus in 2019 presented a unique opportunity to enact this vision and will be the epicentre for DigiFAB’s activities going forward. The White City Campus sits at the heart of the White City Innovation District – a partnership between the College and the London Borough of Hammersmith and Fulham (LBHF). It is home to some of the world’s most innovative organisations, spanning life sciences, deep tech, media and the creative arts.

Imperial’s Molecular Science Research Hub
Imperial’s Molecular Science Research Hub
State-of-the-art facilities there include the Centre for Rapid Online Analysis of Reactions (ROAR) and the Agilent Advanced Measurement Suite.
“The vision of DigiFAB is to bring together experts from multiple fields in one environment, under one umbrella.
Dr Ben Deadman, Facilities Manager at ROAR comments: “The vision of DigiFAB is to bring together experts from multiple fields in one environment, under one umbrella, with the best tools and the best equipment to really tackle those global challenges.
“Tackling these big challenges is impossible if we work in silos; we really do need to collaborate. We need the chemist there to understand the reactions implicitly, we need the engineer to come in and bring their expertise of how to build reactors to make the molecules and then we need the data scientists there to break down that science and turn that into a mathematical relationship."

Imperial’s Centre for Rapid Online Analysis of Reactions (ROAR)
Imperial’s Centre for Rapid Online Analysis of Reactions (ROAR)
DigiFAB aims to accelerate the development of new chemicals and materials important in areas including agriculture, new energy systems, sustainability, and health, including new drugs. This will be delivered through four research ‘pillars’, namely Data and Modelling; Automation; Sensors and Characterisation; and Synthesis and Processes.
Researchers operating in these pillars are already making progress in terms of translating the principles and vision of DigiFAB into practical research.
Degrees of separation

One area where researchers are making important strides in achieving the DigiFAB vision of closed loop material discovery is in the field of separation science. This is vital in a number of areas including water, energy, and the environment. One important example involves separating carbon dioxide gas from either the ambient air around us, or from flue gas from industry (a process known as carbon capture, utilisation and storage (CCUS)). Ideally, we would also like to be able to convert the carbon dioxide captured into useful chemicals through a process known as photoreduction.

The then Business Secretary Alok Sharma MP visited Imperial in 2020 to view the College's Carbon Capture Pilot Plant
The then Business Secretary Alok Sharma MP visited Imperial in 2020 to view the College's Carbon Capture Pilot Plant
Yet, if we are to do this in a way that has an appreciable impact on reducing global emitted carbon, we will need develop materials that can be produced reliably at scale and operate efficiently. Indeed, some figures suggest that the separation industry accounts for around 10-15% of the world’s total energy consumption. With more advanced membrane-based separation, this could be reduced by 90%.
Several DigiFAB researchers are focused on designing, synthesising, characterising and testing advanced porous materials (known as sorbents) that can address various separation challenges. Drs Kim Jelfs, Camille Petit and Becky Greenaway each lead research groups focusing on different aspects of the problem, including computation, automation and synthesis – and how to link them together.
One focus of this work is designing ‘molecular cages’ – chemical structures that contain cavities that can bind smaller molecules within, called ‘guests’. These molecular cages have been shown to accelerate important reactions such as the hydrolysis of amide bonds, degradation of toxins and a range of chemical transformations.

Dr Kim Jelfs, Faculty of Natural Sciences, Department of Chemistry and a Research Fellow at the Royal Society
Dr Kim Jelfs, Faculty of Natural Sciences, Department of Chemistry and a Research Fellow at the Royal Society
However, it can be difficult for researchers to design structures that will be useful as well as successfully synthesised in the lab. This is where a computer-driven approach can be helpful. One technique is to use an evolutionary algorithm that tries to mimic the process of evolution in nature, to explore the molecular space in a highly effective and efficient way.
“Whereas in nature, evolution selects for certain phenotypes and characteristics in organisms, we have molecules and test their ‘fitness’ in terms of their properties,” says Dr Jelfs.
In a recent study, Dr Jelf’s group used a computational approach to explore the effect of introducing asymmetry into the molecular cages. To simplify their synthesis, most cages and their cavities are designed to be highly symmetric. However, this limits the design of cages for potential guest molecules.

An example of the evolutionary molecular cage design
An example of the evolutionary molecular cage design
With the ability to rapidly predict which cages can be readily prepared in the lab, they hope to use this to make novel materials with a wide range of applications in sensing, catalysis, gas storage and drug delivery.
Smart materials on demand

DigiFAB Researchers are also working on the design of advanced two-dimensional (2D) materials that are as thin as a single layer of atoms and have enormous potential in variety of fields from biomedical sensors through to energy storage.
Since the 2004 discovery of graphene – which is composed of a single sheet of carbon atoms – research into this and other two-dimensional (2D) crystal materials has accelerated. Promising new materials such as silicene and borophene (single atomic layers of silicon and boron, respectively) have now been fabricated and their optical and electronic properties investigated.
The role of DigiFAB is to make sure that we have a platform for future industrial players to make best use of the new materials and technologies."
Dr Felice Torrisi, who is DigiFAB co-lead for Sensors and Characterisation, comments:
“If we are able through digitalisation of the production process, to manufacture many of these materials in exactly the same way, repeating their properties in an identical way from one sample to another sample, or indeed thousands or millions of batches - that's exactly what we need in manufacturing. So the role of DigiFAB is to make sure that we have a platform for future industrial players to make best use of the new materials and technologies."

Dr Felice Torrisi, Senior Lecturer in Chemistry of Two-Dimensional Material, Department of Chemistry
Dr Felice Torrisi, Senior Lecturer in Chemistry of Two-Dimensional Material, Department of Chemistry
Dr Torrisi’s group recently developed semi-conducting, printable inks, based on novel two-dimensional (2D) materials, including single-layer molybdenum disulphide (MoS2). They could enable high-performance inkjet-printed electronic circuits, providing a pathway to wearable devices like health monitors, body warmers, radio frequency antennas, and electronic textile displays.

Photo by Alexandre Debiève on Unsplash
Preparing the next generation of change-makers

What is clear to everyone in the field of molecular design is that digital chemistry is here to stay. And so the coming generation of students who go into academic research or industry will need to be well-trained and versed in these techniques. Preparing them for this transition is a major goal for DigiFAB – one which it is encouraging on several fronts.
Back in 2019, Imperial welcomed the first PhD students to the EPSRC Centre for Doctoral Training in Next Generation Synthesis & Reaction Technology. Led by Director Professor Mimi Hii, its mission is to train highly qualified researchers with the ability to collect data using automated, high-throughput reaction platforms, and to apply quantitative and statistical approaches to data analysis and utilisation. This is achieved by incorporating cross-disciplinary skills from engineering, as well as computing, statistics, and informatics into a chemistry graduate programme, which is largely lacking from existing doctoral training in synthetic chemistry.
In October 2021, Imperial launched a new MSc in Digital Chemistry. The one-year programme comprised a series of modules to provide training in the application of data science, machine learning and automation in a diverse range of areas in the chemical sciences. It was designed for on-line/remote delivery, with a one term ‘practical project’ module that can be undertaken either remotely or on campus.
But the DigiFAB vision is for students to begin thinking about the possibilities for digital chemistry at undergraduate level – and irrespective of their chosen degree subject.
In March 2021, DigiFAB and the Faculty of Natural Science Data Science theme delivered a Datathon Challenge. Using the data provided to them, students had to predict the solid-state properties of a material, such as density, crystal symmetry and atom contact distances.
Seventy-five Imperial students and staff participated, with teams from the Departments of Chemistry, Life Sciences, Mathematics, Physics, Bioengineering, Computing and Materials sharing their expertise to find creative solutions. Participants ranged from undergraduates through masters to doctoral students and research associates.
In July 2021, DigiFAB and the Imperial College Advanced Hackspace (ICAH) invited undergraduate students from the Departments of Chemistry and Chemical Engineering to participate in a chemistry hackathon. Teams were tasked with designing and building an automated, low-cost liquid handling platform and turbidimeter, with the final aim of integrating the two systems to work together.

Imperial College Advanced Hackspace bio-lab
Imperial College Advanced Hackspace bio-lab
Indeed, the ICAH now has a bio-lab allowing its diverse community of makers to explore techniques including synthetic biology and molecular fabrication. Recently a team of students who started out in the Hackspace developed a new approach to cancer drug delivery. Typically, chemotherapy drugs are administered directly into veins and circulate freely throughout the body. As well as cancer cells, this inevitably also kills healthy cells, leading to unwanted side effects. Team NanoDIPS’ solution is to package drugs within small nanoscale bubbles that only release their payload in the vicinity of cancer cells. In 2019 the team won second place in an international biomolecular design competition, BIOMOD, hosted in San Francisco.
Dr Kim Jelfs, also a lecturer in computational chemistry at Imperial, comments: “I think this is a really exciting time to be a chemistry student. We've got the equipment, the expertise, and the computer power, and we're already beginning to integrate this throughout the degree programmes. As a computational chemist, I train my students how to use Python Programming Language and they get the opportunity to implement that in building their own characterization and automation equipment in the lab. They're going to come out of here properly trained and ready for the chemical industry.”
Equipped with these skills, the coming generation of change makers has every chance of writing a new chapter in the storied history of chemistry – one that advances civilisation, but in a way that is sustainable, hopefully for millennia to come.
