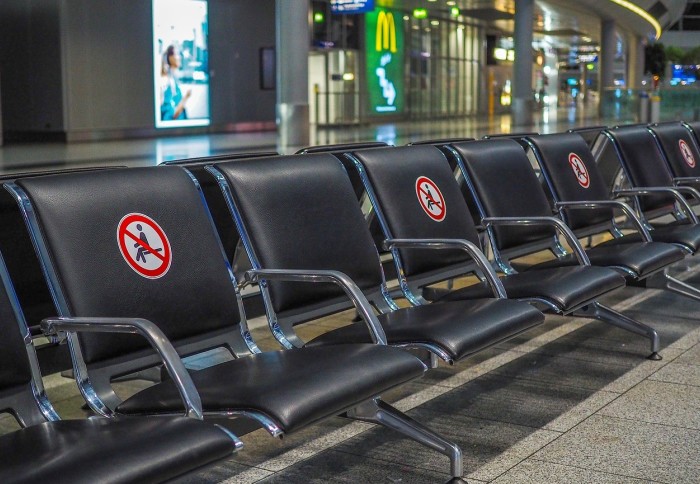
Rapid and cheap COVID-19 detection technologies could be deployed at airports.

Professor Norbert Klein's lab is developing disposable chip-based detectors which can detect COVID-19 in under 10 minutes.
From recent developments in the COVID-19 vaccine roll-out we can be confident that the vaccines can mitigate the virus. But we don’t yet know if the vaccines will lead to eradication, or if the virus will become endemic similar to the flu virus, with new strains emerging annually and immunity waning.
One thing is clear however, the current death toll from COVID-19 is too severe for us to accept it as a part of life like we do with the flu.
Because of this, we can possibly expect continued periodic lockdowns which are widely seen as unsustainable economically, and which have huge implications for education, mental health and general wellbeing.
We know that management of the virus has been quite successful with test and trace systems in East Asia, but for perhaps a myriad of reasons including concerns about government overreach, it hasn’t worked in most Western societies.
I lead the believe that new sensor technologies being developed in labs around the world, including my own laboratory at Imperial, can be a game-changer in managing the virus.
PCR
Current technologies like PCR – the gold-standard – are incredibly useful, but have some inherent limitations which make them not ideal for the socially busy, internationally connected world we live in.
PCR , or Polymerase Chain Reaction to give it its full name, works by reversely transcribing the SARS-CoV-2’s viral genetic material called RNA to DNA, followed by repeatedly copying and pasting the viral DNA until it can be detected. It is highly sensitive, able to work with as few as 100 starting copies of COVID-19 RNA in 1 ml of sample, according to a recent review.
However, there may be an increased false negative rate at these very low detection levels, the process usually takes around 2-3 hours to turnaround results at the best of times, and it requires a healthcare setting or dedicated laboratory environment. This makes PCR not that suitable for checkpoints like airports or other venues which are essential to society functioning as it used to.
My colleague at Imperial, Professor Chris Toumazou, has done some truly fantastic work developing the DnaNudge technology which delivers results in under 90 minutes and can be done anywhere.
Whilst it is a huge improvement removing the need to transport samples to a lab or clinic, 60-90 minutes might still be considered too much friction for deployment at checkpoints.
Ideally, we need tests which turnaround results in a few minutes maximum, which can be completed anywhere (perhaps even at home) and which are cheap (I have heard stories of people paying well over £100 for a test before flying).
I believe that recent developments in graphene-based sensor technology can allow us to do just this.
Graphene
The UK has a strong history of graphene research, with the 2010 Nobel Prize in Physics awarded to Geim and Novoselov at the University of Manchester for the first isolation and characterisation.
The initial hype around the potential of graphene has died down and no clear, major markets have yet been found. Despite this, the technology is developing and graphene foundries have popped up around the world. Graphenea is one such company we partner with, which has developed a scalable process to implement graphene into existing CMOS technology - one of the basic building blocks of modern electronic devices - to produce graphene field-effect transistors (gFETs). These gFETs are the building block of our sensor devices.
So how does this work? You can see what the gFET looks like in the image below. The arrangement, very similar to a classic CMOS transistor, allows us to measure the electrical resistance of the graphene.
We then functionalise the graphene by adding ‘hooks’ to the surface which specifically capture what we want to detect. In our case the hooks have been the antibodies which attack the COVID-19 virus.
When a small volume of sample, such as saliva containing the virus, is introduced to the graphene the antibodies hook the virus particle, and this causes a measurable change in the voltage across the surface thanks to the fact that graphene is a 2D material. The more virus that is hooked, the greater the voltage change.
Figure 1. GFET structure (left). GFTE measures the electrical signal between source and drain; when the virus is captured on the antibody, the electrical signal (resistance) changes as indication of the presence of virus (right).
As good as the gold-standard
The early results are very promising. We first successfully tested detection of the virus spike protein using the antibody hooks, and are now starting to test whole virus particles . The results show strong selectivity, meaning that when no hooks are present you get no detection signals, and when we use antibody hooks for different virus targets we only get very weak signals.
Our early results for sensitivity are also encouraging. Currently we can detect very small concentrations of virus in the attomolar range, which is about 10 virus particles per microlitre. We’re already improving on this by using different hooks called aptamers instead of the antibodies, which have so far achieved an order of magnitude increase in sensitivity, reducing the potential for false negatives. Our graphene-based sensor platform is already comparable to PCR in terms of sensitivity.
Where our platform really stands out is the turnaround time which is under 10 minutes, vs 90 minutes for the best PCR tests.
The big question is how well this works in the real-world. We haven’t yet conducted clinical trials as we only started the work in July. Clinical trials are being discussed for when we have finished optimising the platform.
However, a team in South Korea using very similar technology have conducted a laboratory test using samples taken from a swab, much like you would in a clinical trial. They recently reported in a peer-reviewed journal that the method works very well, also reporting detection turnaround times of 1-2 minutes.
We’re now looking at how this can be made into a device for detection at checkpoints, and how much it would cost.
One drawback is the currently available scale of chip production. Our industry partners suggest that they can scale-up to produce 200,000 chips per day within 6 months if the demand and funding is there, and the cost can be reduced to around 6 euros per chip through reducing chip size. With more work and multiple production facilities opening it really has the potential to become a disposable technology complementing the current PCR tests.
COVID-19 has been a wake-up call highlighting the societal vulnerability to and devastating impacts of infectious disease pandemics. New sensor technologies can play a major role in managing such events, and we can be certain that this isn’t the last pandemic.
Professor Norbert Klein is Chair in Electromagnetic Nanomaterials and Co-Lead of the Electromagnetic and Biochecmical Sensors Network at Imperial College London.
Article text (excluding photos or graphics) © Imperial College London.
Photos and graphics subject to third party copyright used with permission or © Imperial College London.
Reporters
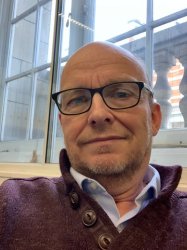
Norbert Klein
Department of Materials

Contact details
Tel: +44 (0)20 7594 6783
Email: n.klein@imperial.ac.uk
Show all stories by this author

Max Swinscow-Hall
Institute for Security Science & Technology