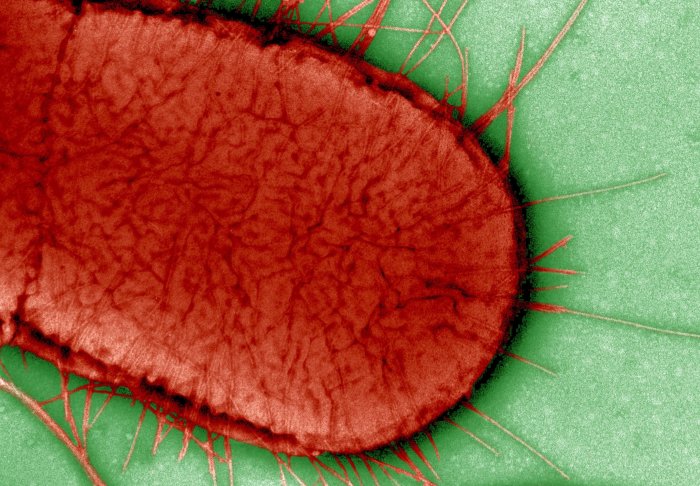
Escherichia coli - or E. coli - is a common bacterium that can cause food poisoning

Fresh insights into how viruses infect bacteria could provide the basis for a new way to tackle antimicrobial resistance (AMR).
New research published this week in PNAS reveals how T7 phage – a virus that targets and infects most strains of E. coli – deploys two key proteins that enable it to take hold of bacteria’s essential cellular machinery.
Significantly, the findings reveal that the previously unexplored protein Gp5.7 is responsible for hijacking forms of a crucial enzyme in E. coli that allow the bacterium to grow and develop resilience in response to changes in its environment.
This new insight into how a phage is optimised to kill bacteria could be used as the foundation for engineering synthetic viruses, capable of combatting many different kinds of drug-resistant infections.
The power of proteins
The study, funded by the Wellcome Trust and led by a multidisciplinary team at the MRC Centre for Molecular Bacteriology and Infection (CMBI), follows a previous investigation that revealed how T7 used proteins in a two-pronged attack against E. coli. Here, researchers discovered that the T7 phage uses the proteins Gp2 and Gp5.7 to zone in on the bacteria’s gene expression machinery (RNA polymerase, or RNAP). This discovery, however, raised an important question: why would a virus develop two proteins to do the same job?
Professor Ramesh Wigneshweraraj explains: “Viruses are quite efficient in many ways: they don’t waste resources.”
“Each gene within a virus will perform a very specific function. However, this study seemed to indicate an element of redundancy: it appeared that the T7 virus was producing two different proteins to do the same thing. That prompted us to think that there was something more to it.”
With the role of the first protein, Gp2, already well established, the team set about pinpointing the function performed by the lesser-explored Gp5.7.
They discovered that while both Gp2 and Gp5.7 inhibit the RNAP responsible for expressing E. coli’s ‘housekeeping’ genes – the key genetic elements that allow a bacterium to function normally – Gp5.7 performs an additional biological role in attacking the form of RNAP essential to stress resistance, adaptation and virulence gene expression. As a consequence, the E. coli bacterium becomes unable to face up to new challenges in its environment.
Above: The left-hand Petri dish shows how wild-type T7 phage kills E. coli; on the right mutant T7 phage without Gp5.7 is unable to effectively kill E. coli.
Synthetic phages vs phage therapy
Equipped with an improved understanding of how viruses such as T7 phage infect their prey, the team behind the study are now looking to develop synthetic phages capable of tackling a broad range of bacteria.
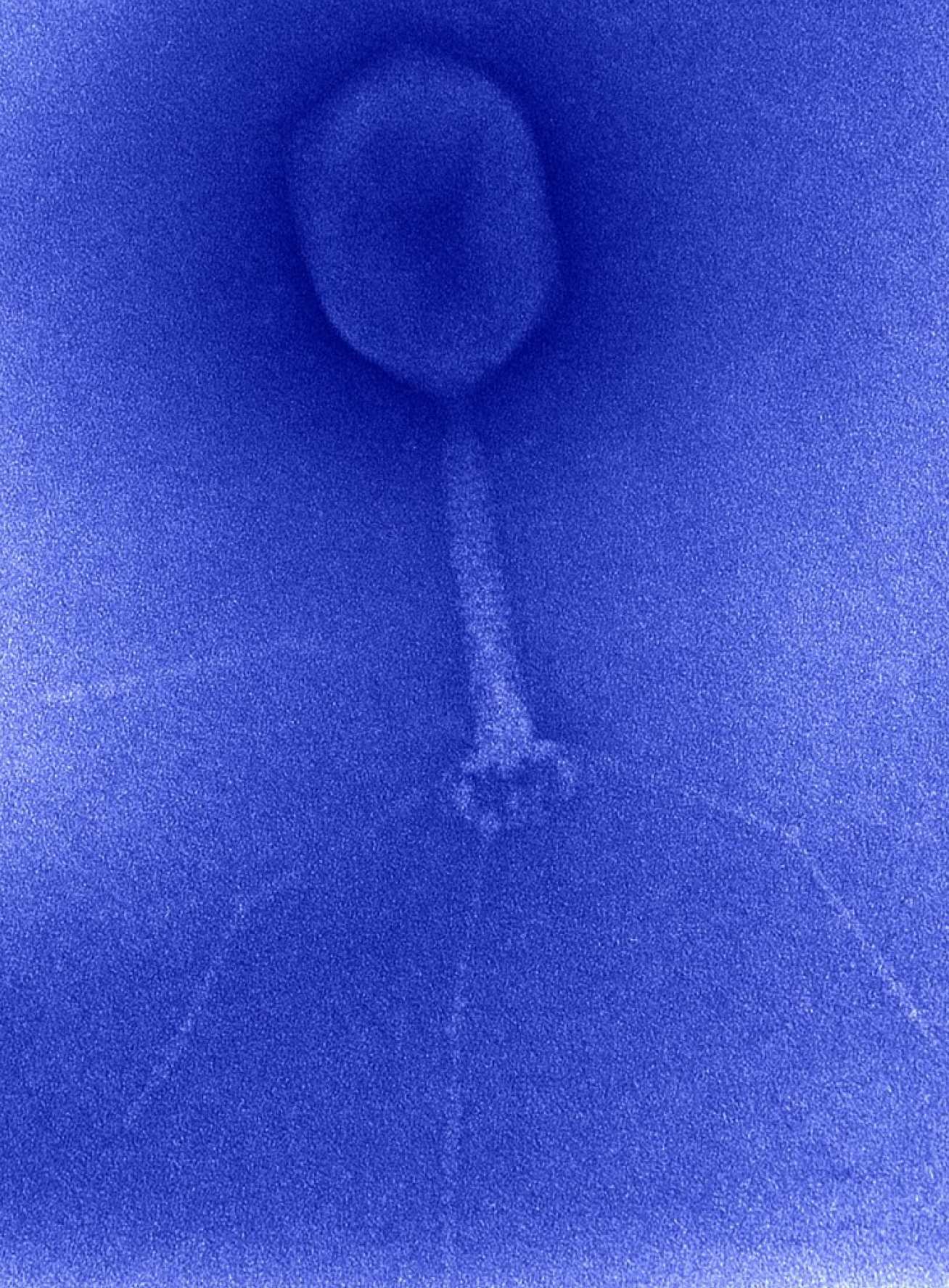
“From our work over the past several years, we’ve uncovered different kinds of toxic cargo in viruses. The difficulty is in finding a way to deliver them into bacteria in order to treat infections,” comments Professor Wigneshweraraj.
“A synthetic phage is one option, but there are other possible modes of delivery that we are interested in exploring.”
Lead author Dr Aline Tabib-Salazar explains why this could be a more successful approach than phage therapy, which is being increasingly used to treat drug-resistant bacterial infections: “The limitation of phage therapy is that phages are highly specific in terms of what kinds of bacterial strains they can infect; they can’t be considered broad spectrum.”
“Another serious issue is that phage therapy can still leave behind bacteria that are capable of passing on toxic effects or developing resistance.”
“Our proposed approach overcomes this limitation by taking toxic cargoes from several different phages, putting them into a synthetic chassis, and engineering it so that it can infect many different kinds of bacteria,” adds Professor Wigneshweraraj.
“It’s also worth noting that phage therapy has been used previously to treat bacterial infections in plants and animals, so there is also the potential that a synthetic phage could be applied in other contexts outside of the human body.”
The bigger picture: meeting the challenge of AMR
Antimicrobial resistance – a microorganism’s ability to stop an antimicrobial working against it – has become an increasingly serious global health issue in recent years. The rise of superbugs has been accelerated by the misuse and overuse of antimicrobial treatments, resulting in the spread of drug-resistant strains of many common infections. In 2016, just under half a million people developed multi-drug resistant tuberculosis, and there is evidence that AMR is beginning to have a serious impact on how other conditions (such as malaria, HIV and influenza) are treated too.
In 2015, WHO member states backed a global action plan to tackle AMR, a key objective of which is to increase investments in new medicines and treatment. Consequently, research institutes, centres and networks around the world – including Imperial College’s Antimicrobial Research Collaborative (ARC) – are now working urgently to identify innovative new strategies and treatments to tackle drug-resistant superbugs.
A key part of the fight against AMR lies in the field of molecular bacteriology. There is a need to better understand the changes that make bacteria resistant, and to map bacterial cellular processes in such a way that new treatment targets can be identified. By providing a vital part of the puzzle to understanding the specific strategies phages use when attacking bacteria, these newly-published insights into T7 phage could have applications that extend far beyond E. coli.
Commenting on the wider significance of the findings, Professor Wigneshweraraj says: “With this study, we’ve substantially advanced our understanding of a bacterial-viral relationship, which in turn will better equip us to address the issue of antimicrobial resistance. Our findings can be used as a starting point to ask many other fundamental questions in bacteriology.”
Professor Steve Matthews adds: “Our understanding of how phages inhibit bacterial gene expression processes is progressing rapidly, and our structural and molecular insights presented here provide essential knowhow for the design new antibacterial therapeutic strategies.”
‘T7 phage factor required for managing RpoS in Escherichia coli’ by Aline Tabib-Salazar & Bing Lui, et al, is published in Proceedings of the National Academy of Sciences of the United States of America.
Image credit: 'Bacteriophage, TEM' and 'Electron micrograph of Escherichia coli, close-up' by David Gregory & Debbie Marshall.
Supporters
Article text (excluding photos or graphics) © Imperial College London.
Photos and graphics subject to third party copyright used with permission or © Imperial College London.
Reporter

Ms Genevieve Timmins
Academic Services

Contact details
Email: press.office@imperial.ac.uk
Show all stories by this author
Leave a comment
Your comment may be published, displaying your name as you provide it, unless you request otherwise. Your contact details will never be published.