Numerical analysis of thermo-active retaining structures
BACKGROUND
The use of ground source energy systems (GSES) is a well-established method to provide low carbon heating and cooling to buildings, diversify the energy mix and help meeting increasingly stricter sustainability targets. Shallow geothermal energy can be exploited by means of different types of systems either installed directly within the ground (closed and open loop systems) or embedded within geotechnical structures (such as foundation piles, tunnel linings, basement slabs and retaining walls). The latter systems may often be more economical as limited additional works are required since these structures are needed to provide stability. Furthermore, they generally have a large contact area with the ground, which improves the thermal performance. However, the use of geotechnical structures as heat exchanger poses new challenges to geotechnical engineers since any exchange of heat will necessarily result in changes in temperature of the thermo-active structure and surrounding soil, inducing additional loads within the structures as well as changes of mechanical and hydraulic conditions within the soil mass. A simplified framework for understanding the behaviour of thermo-active piles when subjected to thermal loads has been developed based on the field observations (Bourne-Webb et al., 2009; Amatya et al., 2012; Bourne-Webb et al., 2013); however, limited research has been carried out on the thermo-mechanical behaviour of thermo-active retaining walls, both in terms of field studies – only one published work by Brandl (2006) – and numerical studies. Thus, many uncertainties regarding the safe design and operation of these structures exist, as they behave in a very different manner when compared to piles due to their more complex geometry and boundary conditions.
RESEARCH AIMS
The aim of the research is to provide insight into the long-term behaviour of thermo-active retaining walls, with the objective of outlining design guidelines for these structures, both from a perspective of energy efficiency and structural safety. This is to be accomplished by carrying out detailed computational studies of non-isothermal soil-structure interaction problems using ICFEP – Imperial College Finite Element Program (Potts and Zdravković, 1999), which is capable of performing fully coupled thermo-hydro-mechanical analyses (Cui et al., 2018). The main objectives of this study are:
• Understand the fundamental mechanisms of soil-structure interaction and how these affect the response of thermo-active retaining walls;
• Explore appropriate modelling techniques of thermo-active retaining walls in 2D by comparing to 3D analyses;
• Assess the impact of long-term cyclic thermal loading on the stability and serviceability of thermo-active retaining walls;
• Provide recommendations and guidelines for design
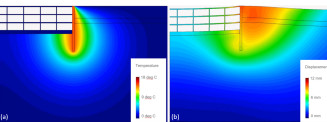
Figure 2: Numerical simulation of a retaining wall problem (a) temperature contours and (b) displacement contours
REFERENCES
Amatya, B., Soga, K., Bourne-Webb, P. J., Amls, T. & Laloui, L. (2012) Thermo-mechanical behaviour of energy piles. Geotechnique, 62.
Banks, D. (2012) An Introduction to Thermogeology: Ground Source Heating and Cooling. 2nd Edition.Wiley-Blackwell, Chichester.
Bourne-Webb, P. J., Amatya, B., Soga, K., Amis, T., Davidson, C. & Payne, P. (2009) Energy pile test at Lambeth College, London: geotechnical and thermodynamic aspects of pile response to heat cycles. Geotechnique, 59, 237-248.
Bourne-Webb, P. J., Soga, K. & Amatya, B. (2013) A framework for understanding energy pile behaviour. Proceedings of the ICE - Geotechnical Engineering, 166, 170-177.
Brandl, H. (2006) Energy foundations and other thermo-active ground structures. Géotechnique, 56 (2), 81-122.
Cui, W., Potts, D.M., Zdravković, L., Gawecka, K.A., Taborda, D.M.G. (2018) An alternative coupled thermo-hydro-mechanical finite element formulation for fully saturated soils, Computers and Geotechnics, 94: 22-30.
Potts, D.
Contact Geotechnics
Geotechnics
Civil and Environmental Engineering
Skempton Building
Imperial College London
South Kensington Campus
London, SW7 2AZ
Telephone:
+44 (0)20 7594 6077
Email: j.otoole@imperial.ac.uk
Alternatively, view our people lists
Follow us on Twitter: @GeotechnicsICL
We are located in the Skempton Building (building number 27 on the South Kensington Campus Map). How to find us