Research Images
Polymer Electrolyte Membrane Fuel Cells
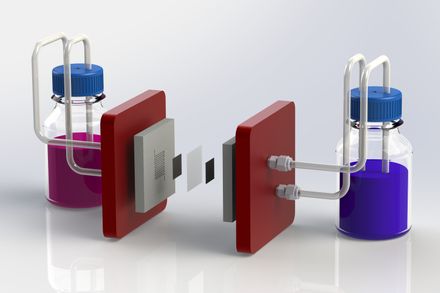
Redox Flow Batteries
(Some) Current Areas of Research
Fuel cells: a type of electrochemical energy conversion device, used to transform a chemical fuel and an oxidising agent (typically hydrogen and oxygen, respectively) into electricity, through a pair of redox reactions.
e.g. Acidic H2 Fuel Cell: (Anode) H2 -> 2H+ + 2e- (Cathode) 1/2O2 + 2H+ + 2e- -> H2O |
e.g. Alkaline H2 Fuel Cell: (Anode) H2 + 2OH- -> 2H2O + 2e- (Cathode) 1/2O2 + H2O + 2e- -> 2OH- |
---|
Our group performs work on low temperature (PEM, polymer electrolyte membrane) fuel cells including proton and hydroxide conducting membrane materials1. Work is associated with understanding the electrocatalysis of the oxygen reduction and hydrogen oxidation reactions at the catalyst level through advanced electrochemical modelling2-5. In concert with this we are also interested in understanding the limitations imposed by mass transport of reactants through the catalyst layer. We have developed a range of new approaches to characterise fuel cells4,6-11. These aspects all come together in studies associated with reducing the loading of platinum group metals in the layers through either reduced loading electrodes or non-precious metal electrocatalysts. Finally, issues associated with degradation of fuel cell components12 through corrosion or poisoning of catalysts are considered along with amelioration approaches for allowing fuel cells to operate with impure fuels13 or in the presence of impure air14,15. Work in our group has been spun out into the company Bramble Energy which considers production of fuel cells (and other electrochemical devices) using printed circuit board technology16-18.
- Anion-exchange membranes in electrochemical energy systems - Varcoe JR, et al., Energy Environ. Sci., 2014, 7, 3135-3191.
- Optimizing Oxygen Reduction Catalyst Morphologies from First Principles - Ahmad EA, et al., J. Phys. Chem. C, 2015, 119 (29), 16804–16810.
- General Models for the Electrochemical Hydrogen Oxidation and Hydrogen Evolution Reactions: Theoretical Derivation and Experimental Results under Near Mass-Transport Free Conditions - Kucernak AR, Zalitis C, J. Phys. Chem. C, 2016, 120 (20), 10721-10745.
- A catalyst layer optimisation approach using electrochemical impedance spectroscopy for PEM fuel cells operated with pyrolysed transition metal-N-C catalysts - Malko D, et al., J. Power Sources, 2016, 323, 189-200.
- Performance measurements and modelling of the ORR on fuel cell electrocatalysts - the modified double trap mode - Markiewicz M, et al., Electrochim. Acta, 2015, 179, 126-136.
- What Happens Inside a Fuel Cell? Developing an Experimental Functional Map of Fuel Cell Performance - Brett DJL, et al., ChemPhysChem, 2010, 11, 2714-2731.
- Investigation of convective transport in the gas diffusion layer used in polymer electrolyte fuel cells - Beruski O, et al., Phys. Rev. Fluids, 2017, 2, 103501.
- Assessing the performance of reactant transport layers and flow fields towards oxygen transport: A new imaging method based on chemiluminescence - Lopes T, et al., J. Power Sources, 2015, 274, 382-392.
- Thin solid state reference electrodes for use in solid polymer electrolytes - Smith G, et al., Electrochem. commun., 2014, 43, 43-46.
- Advanced Diagnostics Applied to a Self-Breathing Fuel Cell - Obeisun OA, et al., ECS Trans., 2014, 61, 249-258.
- Spatially resolved diagnostic methods for polymer electrolyte fuel cells: a review - Kalyvas C, et al., WIREs Energy Environ., 2014, 3, 254-275.
- Influence of microporous layer on corrosion of metallic bipolar plates in fuel cells - Castanheira L, et al., J. Power Sources, 2019, 418, 147-151.
- Gas phase recovery of hydrogen sulfide contaminated polymer electrolyte membrane fuel cells - Kakati BK, Kucernak ARJ, J. Power Sources, 2014, 252, 317-326.
- Recovery of Polymer Electrolyte Fuel Cell exposed to sulphur dioxide - Kakati BK, et al., Int. J. Hydrog. Energy, 2016, 41, 5598-5604.
- Using corrosion-like processes to remove poisons from electrocatalysts: a viable strategy to chemically regenerate irreversibly poisoned polymer electrolyte fuel cells - Kakati BK, et al., Electrochim. Acta, 2016, 222, 888-897.
- Anode ink formulation for a fully printed flexible fuel cell stack - Hakola L, et al., Flex. Print. Electron., 2020, 5, 025002.
- Development of open-cathode polymer electrolyte fuel cells using printed circuit board flow-field plates: Flow geometry characterisation - Obeisun OA, et al., Int. J. Hydrog. Energy, 2014, 39, 18326-18336.
- Current collector design for closed-plenum polymer electrolyte membrane fuel cells - Daniels FA, et al., J. Power Sources, 2014, 249, 247-262.
Electrolysis is the electrochemical process of water splitting and is the key method of green hydrogen production.
Our water electrolysis research focuses on a number of different aspects, including:
- Synthesis of new, highly active IrOx and doped IrOx catalysts.
- Synthesis of non-precious metal hydrogen evolution (HER) catalysts.
- Ultra-low loading, high mass transport, floating electrode testing of oxygen evolution and hydrogen evolution catalysts.
- Anion exchange membrane (AEM) water electrolysis.
- Polymer electrolyte membrane (PEM) water electrolysis to produce green hydrogen with the co-production of a second valuable chemical such as ozone or hydrogen peroxide.
Redox flow batteries are promising candidates for large scale energy storage due to their versatile architectural design. They consist of an electrochemical cell, fed by external electrolyte storage tanks.
We develop new chemistries and battery components devoted to reducing RFB capital costs and environmental footprint. This research spans a wide range of electrolyte-electrolyte, electrolyte-gas, inorganic and organic chemistries.
Work carried out in this area (utilsing H2-Mn chemistry, in the form of a regenerative fuel cell) led to the spin out of RFC Power - who are perfecting, scaling and marketing this technology for large scale energy storage applications. We continue to collaborate with RFC, to develope this further.
The concept of electrochemical hydrogen compression is reasonably straightforward – a device, which requires no moving parts, combines both purification and compression in a single electrochemical process. The system, which is not dissimilar to other electrochemical devices, is supplied with an impure and/or lower pressure hydrogen gas stream at the anode, where the gas is oxidised at a catalyst surface. The resulting protons are driven across a proton-specific exchange membrane (PEM) to the cathode, where they are reduced back to a pure stream of hydrogen gas (up to 1000 bar).
Our H2 Electrochemical Pump research focuses on hydrogen purification from impure gas streams containing methane, carbon monoxide, carbon dioxide, other low-mw hydrocarbons, and hydrogen sulphide. These impurities would typically be present in hydrogen produced from steam methane reforming or in natural gas blending.
Poison mitigation strategies for the use of impure hydrogen in electrochemical hydrogen pumps and fuel cells - Jackson C, Raymakers L, Mulder M, Kucernak A, et al., J. Power Sources, 2020, 472, 228476.
-
The Floating Electrode.
The floating electrode allows the evaluation of electrocatalysts for fuel cell and electrolyser reactions without the apparent mass-transport limitations of other methods such as the rotating disc electrode (RDE). This method typically examines ultra-low catalyst loadings (0.1-10 µgPt cm-2), and can identify electrochemical characteristics not visible via traditional techniques.
Electrocatalytic performance of fuel cell reactions at low catalyst loading and high mass transport - Zalitis CM, Kramer D, Kucernak AR, Phys. Chem. Chem. Phys., 2013, 15, 4329-4340.
-
The Gas Accessible Membrane Electrode (GAME).
The GAME is a complete electrode configuration providing a well-defined three-phase interface designed to facilitate rapid, two-way, mass transport – similarly, with a rate constant 1-2 orders of magnitude larger than other techniques. Due to the system design, the electrochemical data can be complemented further with coupled, on-line and in situ analyses (such as mass spectrometry, infrared spectroscopy, etc.), lending itself to studying complex electrochemical conversion process such as carbon dioxide reduction.
Gas Accessible Membrane Electrode (GAME): A Versatile Platform for Elucidating Electrocatalytic Processes Using Real-Time and in Situ Hyphenated Electrochemical Techniques – Zhang G, Kucernak A, ACS Catal., 2020, 10 (17), 9684–9693.
One of the biggest challenges facing the wide-scale deployment of PEMFCs is considered to be the requirement for precious metal catalysts. Traditionally, platinum (and alloys thereof) are used on both sides of the cell (HOR and ORR reactions). While one research aim is to optimise these catalysts, thereby reducing the quantity needed per cell, another is to remove this reliance entirely.
For the Oxygen Reduction Reaction (ORR): single atom, non-precious group metal catalysts (NPGMs) are one of the most promising catalyst candidates to date. These generally take the form of pyrolysed transition metal complexes supported on carbon materials, M-Nx/C.
Recent work has explored the use of Fe-N/C materials identifying particularly high performance in single atomic catalysts synthesised by the exchange of iron into a preformed carbon-nitrogen matrix. These materials have a high density of these atomic active sites (7 wt%Fe vs. the typical 1-3 wt%Fe) and display excellent ORR activity in both RDE and device testing.
High loading of single atomic iron sites in Fe–NC oxygen reduction catalysts for proton exchange membrane fuel cells – Mehmood A, Gong M, Jaouen F, et al., Nat. Catal., 2022, 5, 311–323.
Besides Fe-N/Cs, other transition metals show promising performance, and we are also exploring the potential for NPGM catalysts in other applications.
Recent Talks/Interviews
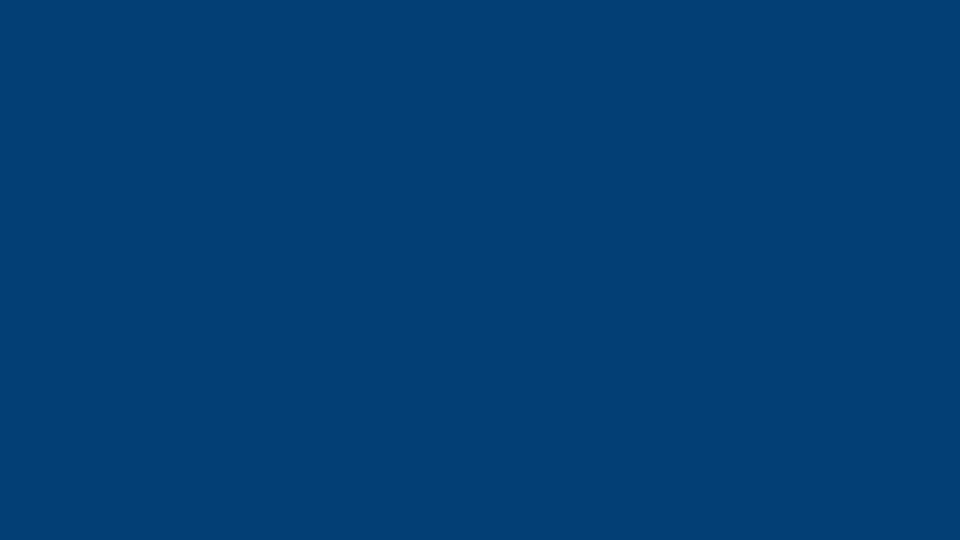
2023-05-23: Colleen Jackson, IDRIC
Dr Colleen Jackson discussing green hydrogen and the co-production of an alternative oxidant.
Interview with Dr Colleen Jackson, who works on the IDRIC project: MIP 7.2, about research into 'electrolysis for green hydrogen and co-produced chemicals at scale'.
More information on her project can be found on the IDRIC website: IDRIC MIP7.2
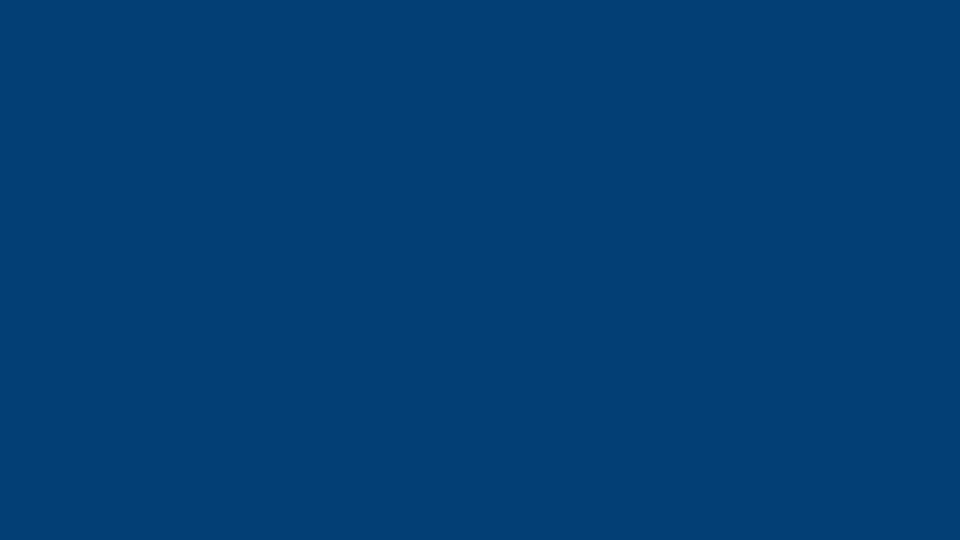
2023-04-17: Anthony Kucernak, Eko zona HRT
Prof. Anthony Kucernak discussing hydrogen and fuel cells on Croatian TV.
Interview with Professor Anthony Kucernak discussing the role of hydrogen and fuel cells in the transition towards renewable energy.
Anthony was interviewed on Croatian television program 'Eko zona' (Eco-zone).
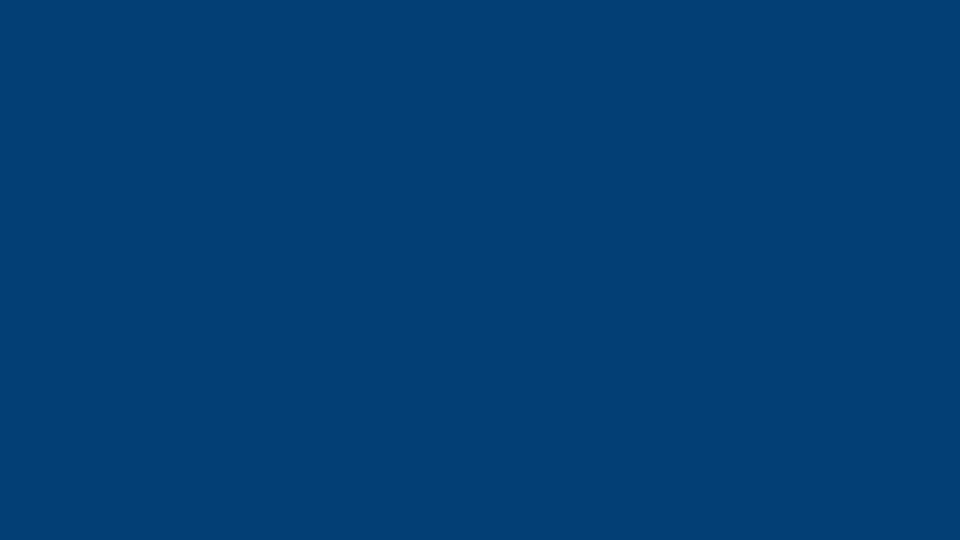
2023-03-30: Anthony Kucernak, XII RCGI Colloquium
Prof. Anthony Kucernak discussing the storage of renewable energy at scale (RFBs and H2).
Professor Anthony Kucernak presenting the 'Storage of renewable energy at scale - the role of flow batteries and hydrogen'.
Anthony presented at the XII RCGI Colloquium: 'Energy storage at large scale for the energy transition and beyond' arranged by the Research Centre for Greenhouse Gas Innovation.
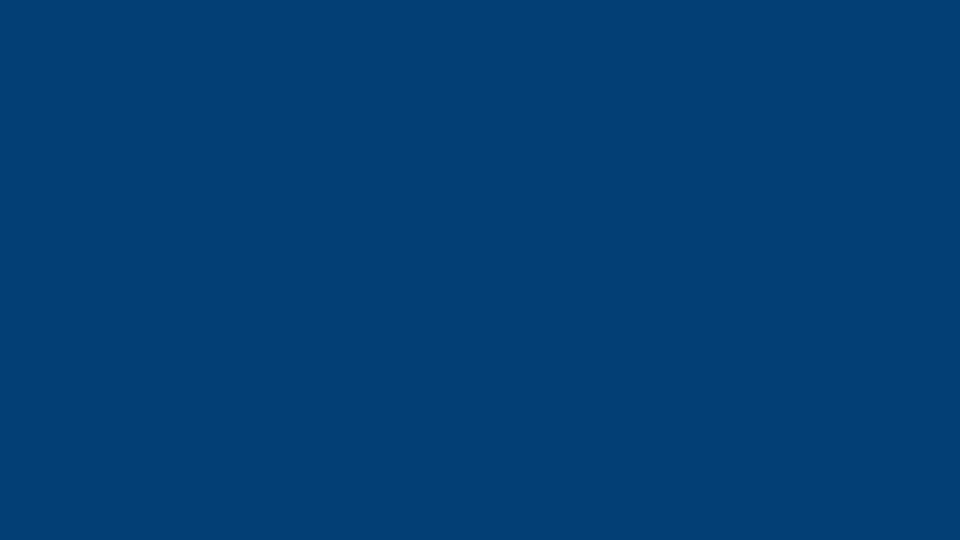
2021-11-03: Anthony Kucernak, RSC Panel Discussion
Prof. Anthony Kucernak joins an RSC panel discussion: the chemistry of hydrogen production and use.
Professor Anthony Kucernak joins Royal Society of Chemistry (RSC) panel discussion on 'The Chemistry of Hydrogen Production and Use'.
This panel, held in the run up to COP26, discusses the replacement of fossil fuels in power, transport and industry. (More info can be found on the RSC website: 'Chemistry and COP26'.)
Chair - Sue Nelson
Prof Anthony Kucernak, Imperial College London
Prof Francis Livens, University of Manchester
Prof Edman Tsang, University of Oxford
Dr Sam French, Johnson Matthey
Fiona Landy, University of St Andrews
Links
Contact Details
Prof. Anthony Kucernak
G22B
Molecular Sciences Research Hub (MSRH)
Imperial College London
White City Campus
London
W12 0BZ
United Kingdom
Phone: +44 (0)20 7594 5831
Fax: +44 (0)20 7594 5804
Email: anthony@imperial.ac.uk