The recovery and re-use of low-grade waste heat and effective utilisation of renewable resources for heat and power provision are essential components of a sustainable energy future. In the UK, for example, natural gas consumption for heating purposes is double that of electricity, while in the domestic sector the demand for heat is currently somewhere between 3 and 5 times that of electricity. Nevertheless, the energy debate focuses mainly on the latter. The availability of most alternative energy sources (e.g., solar, wind) exhibits significant fluctuations (caused by day-night cycles, weather, etc.), while the consumption also fluctuates considerably, according to users’ needs. The resulting mismatch between renewable energy supply and demand gives rise to a need for reliable and efficient energy storage systems to smooth out the imbalance. A disparity between supply and demand is also an important factor that arises when considering the opportunities for low-grade waste heat utilisation, which is abundantly available in the industrial and commercial sectors. A similar problem occurs in the domestic environment, especially when considering ‘over the fence’ solutions which offer an improved performance, both from an investment and an emission reduction perspective, over the self-contained re-use and/or conversion of heat within individual plants.
The research activities conducted by the Energy Division are:
- Waste heat recovery and thermodynamic conversion
- Solar heating, cooling and power
- High-temperature high-efficiency hybrid PV-thermal solar systems
- Low-carbon heating technologies
- Large-scale electricity storage
- Thermal energy storage
Energy Division Content
1. Waste heat recovery and thermodynamic conversion
The Energy Division team focuses on technologies for thermal energy conversion and utilisation; in particular low- to medium-grade heat from renewable energy sources (solar and geothermal) and the waste heat from industrial processes. Many industrial processes produce immense amounts of thermal energy, albeit at low- to medium-temperatures for which the Carnot efficiency that limits conversion to power is inherently low. The conversion of the thermal energy into electricity can be achieved by means of Organic Rankine Cycle (ORC) systems and supercritical CO2 cycle (S-CO2) systems, which are capable of converting this low- to medium-grade heat more efficiently compared to conventional steam Rankine cycle systems.
This research aims to investigate how to make ORC, S-CO2 cycle and combined systems capable of converting the low- to medium-grade heat more efficiently. The main focus is to determine suitable working fluids, to develop efficient components (e.g., heat exchanger, expander, etc.) and to propose innovative system configurations. In particular, computer-aided molecular-design (CAMD) frameworks have been developed, which introduce the working fluid as an unknown element to the system optimisation. This leads to the possibility of combining systematically the selection or design of the working fluid with system design and operational parameter optimisation, thereby omitting the subjective fluid pre-selection of conventional approaches. Moreover, the use of novel working fluid mixtures and additives in ORC and S-CO2 cycle systems can provide a better thermal match and reduce exergy losses from the heat transfer processes, thereby improving thermal efficiency and power output. Work on expanders focuses on the use of volumetric machines, such as reciprocating piston expanders, as alternatives to conventional turbines in small-scale applications. Heat exchangers with coatings, patterned or structured surfaces, and integrated thermal storage are designed and developed to allow smaller, more affordable devices with prolonged high-efficiency near-design-point operation. Various novel system configurations as well as combined systems are also investigated for diverse wide-range applications including solar, geothermal and waste-heat recovery.
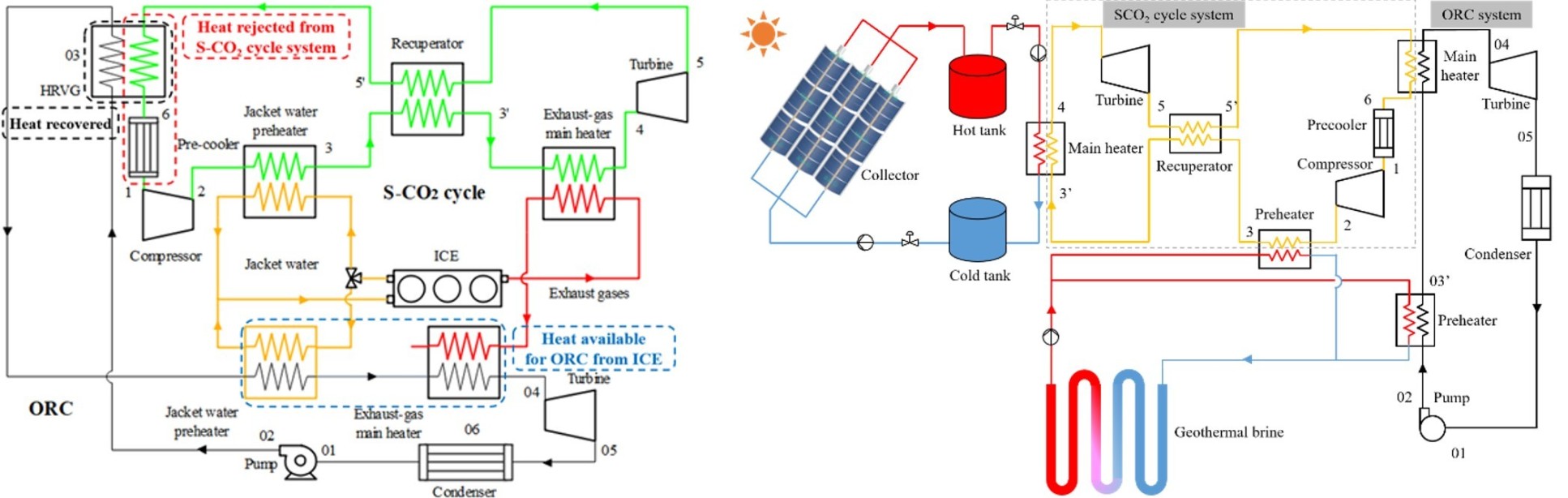
Alternative heat engines for the conversion of low-grade heat to useful work are also being researched. The Energy Division is involved in the European Up-THERM project, which is aiming to provide an affordable alternative to conventional heat engine prime-movers for combined heat and power (CHP) applications (for more information see the Up-THERM project summary).
The CEP group is also involved in the research activities of the UNIHEAT project under Theme 4 - Thermodynamic power generation cycles for improved energy efficiency. UNIHEAT aims to increase the energy efficiency of several processes in oil refineries in addition to upstream and downstream operations (for more information see here).
The group is also leading the “Energy-Use Minimisation via High Performance Heat-Power-Cooling Conversion and Integration: A Holistic Molecules to Technologies to Systems Approach” project (iHPC). The project is a four-year multidisciplinary project funded by the Engineering and Physical Sciences Research Council (EPSRC) aimed at minimising primary-energy use in UK industry with next-generation technological solutions for waste heat recovery and conversion to power or cooling.
Selected publications:
[1] Wang Y, Song J, Chatzopoulou MA, Sunny N, Simpson MC, Wang J, Markides CN. A holistic thermoeconomic assessment of small-scale, distributed solar organic Rankine cycle (ΟRC) systems: Comprehensive comparison of configurations, component and working fluid selection. Energy Conversion and Management 2021; 248: 114618. https://doi.org/10.1016/j.enconman.2021.114618
[2] Song J, Li X, Wang K, Markides CN. Parametric optimisation of a combined supercritical CO2 (S-CO2) cycle and organic Rankine cycle (ORC) system for internal combustion engine (ICE) waste-heat recovery. Energy Conversion and Management 2020; 218: 112999. https://doi.org/10.1016/j.enconman.2020.112999
[3] Song J, Loo P, Teo J, Markides CN. Thermo-economic optimization of organic Rankine cycle (ORC) systems for geothermal power generation: A comparative study of system configurations. Frontiers in Energy Research 2020; 8: 6. https://doi.org/10.3389/fenrg.2020.00006
[4] White MT, Oyewunmi OA, Chatzopoulou MA, Pantaleo AM, Haslam AJ, Markides CN. Computer-aided working-fluid design, thermodynamic optimisation and thermoeconomic assessment of ORC systems for waste-heat recovery. Energy 2018; 161: 1181-98. https://doi.org/10.1016/j.energy.2018.07.098
[5] Chatzopoulou MA, Markides CN. Thermodynamic optimisation of a high-electrical efficiency integrated internal combustion engine–Organic Rankine cycle combined heat and power system. Applied Energy 2018; 226: 1229-51. https://doi.org/10.1016/j.apenergy.2018.06.022
[6] White MT, Oyewunmi OA, Haslam AJ, Markides CN. Industrial waste-heat recovery through integrated computer-aided working-fluid and ORC system optimisation using SAFT-γ Mie. Energy Conversion and Management 2017; 150: 851-69. https://doi.org/10.1016/j.enconman.2017.03.048
[7] Oyewunmi OA, Markides CN. Thermo-economic and heat transfer optimization of working-fluid mixtures in a low-temperature organic Rankine cycle system. Energies 2016; 9:448. https://doi.org/10.3390/en9060448
[8] Markides CN. The role of pumped and waste heat technologies in a high-efficiency sustainable energy future for the UK. Applied Thermal Engineering 2013; 53: 197-209. https://doi.org/10.1016/j.applthermaleng.2012.02.037
2. Solar heating, cooling and power
The IEA projects that solar energy has the potential to cover one-third of the world’s energy consumption by 2060. Solar-thermal technologies in particular can make a significant contribution to primary fuel and emissions reduction targets, and energy security. A key challenge is improving efficiency and economics for regions of low irradiance and for small-scale applications for regions where land area is limited. Solar thermal collectors are a mature technology for the conversion of the solar irradiance into thermal energy for industrial, commercial and domestic applications. Low-temperature thermodynamic cycles for thermal energy conversion, such as organic Rankine cycle (ORC) technology, offer the possibility of using simple and affordable non-concentrating solar collectors in small systems. Alternatively, the solar irradiance can be converted directly into electricity by means of solar PV cells.
Photovoltaic (PV) cells for the direct conversion of solar radiation to electricity also produce a significant amount of low-grade heat. The solar to electricity conversion efficiency of PV cells can be improved by cooling down the cells with a cooling medium. The low-grade heat obtained from such a hybrid PV/thermal collector (PV/T) can be used in domestic heating applications or employed for cooling via an absorption refrigeration cycle (Section 2.1).
The Energy Division is interested in the modelling of the solar ORC as part of a versatile, co-generation (heating and power) or tri-generation (heating, cooling, and power) system. Such a system has potential for domestic applications, where they can be integrated with conventional solar hot-water systems at low cost (Section 2.2).
Solar-thermal collectors provide an opportunity for low-cost sustainable heat provision for domestic, commercial, and industrial applications. Research is undertaken on the techno-economic performance of different systems and their value in a whole-energy system context (Section 2.3).
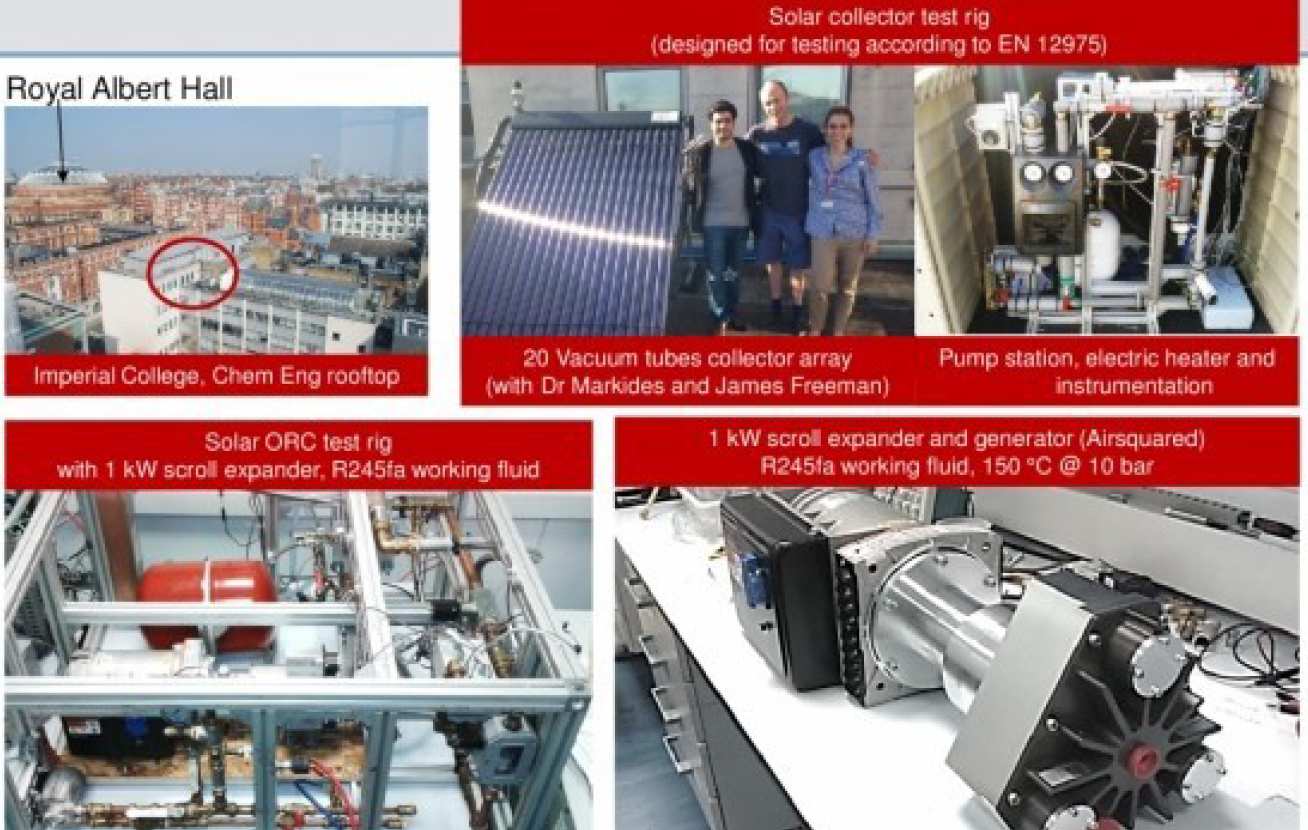
2.1. Solar cooling
The CEP laboratory is currently investigating the use of thermally-powered absorption refrigeration systems in solar cooling and combined cooling, heat and power (CCHP or trigeneration) systems. Thermally-powered refrigeration technologies such as the ammonia-water Diffusion Absorption Refrigeration (DAR) cycle can be easily integrated with solar thermal collectors to provide solar-powered cooling. The DAR cycle exploits the principle of partial pressure to provide cooling, through the use of an auxiliary gas in the evaporator (typically hydrogen or helium). DAR systems use bubble-pumps for fluid circulation (hence have no moving parts) and can be manufactured as single-piece units suitable for small-scale (< 1kW output) low-cost applications. The CEP laboratory indoor experimental facilities are used to study the thermodynamics of the DAR cycles and to optimise the charging parameters such as mixture pairs, mixture concentration and pressure.

The CEP Laboratory is developing a universal model that predicts a DAR cycle performance in steady-state (waste heat) and transient (solar) energy input conditions. Outdoor experimental facilities are mounted on the roofs of Roderic Hill and Huxley buildings in Imperial College London to study the possibility of using solar modules as a heat source to drive a DAR unit for refrigeration and ice production.
2.2. Solar organic Rankine cycle
A solar ORC system uses an organic working fluid instead of water/steam as in the conventional Rankine power-cycle. The thermal energy needed to evaporate the working fluid is provided by solar thermal collectors. The heat rejected during de-superheating and condensation may also be used for useful downstream processes such as water heating.
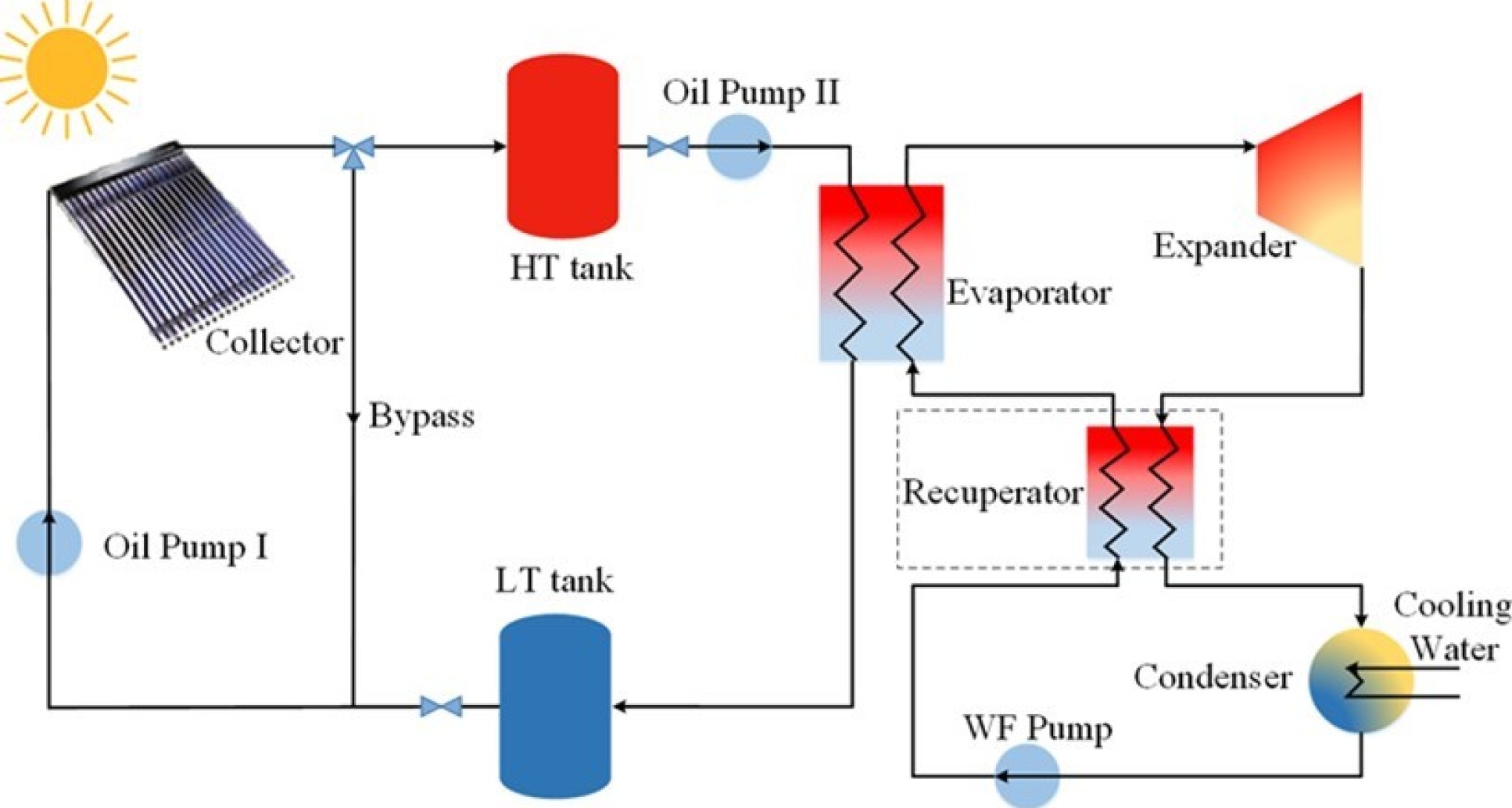
Modelling and optimisation
The performance of renewable energy (and particularly solar power) systems can vary significantly with location. In order to optimise the design of a solar combined heat and power (SCHP) system, a dynamic system model was constructed, which allows the various system configurations considering different working fluids, components and cycles to be simulated under realistic climate conditions. The results of the annual simulation are used to select the optimal design and operating parameters for the solar ORC system.
Experimental system
An ORC rig was constructed in the CEP lab in order to characterise the performance of all system components over a wide range of operating conditions. Heat input is provided by an electrical oil heater, mimicking the thermal output of a solar thermal collector array installed on the roof of the Department.
2.3. Solar thermal heating
Solar-thermal collectors gather heat from sunlight, which can then be used for space heating or hot water provision as well as low-temperature industrial processes. It is a mature technology that has been around for decades, and recent pushes towards sustainable, low-emission energy systems make them solar-assisted heating more and more attractive. Two main collector types are available on the market. Flat-plate collectors consist of a flat absorber plate thermally connected to pipes through which heat transfer fluid flows. The collectors are thermally insulated and covered with a glazing layer to reduce heat losses. Flat-plate collectors can efficiently provide heat at temperatures up to about 100 °C. The second type are evacuated-tube collectors, where the collector is placed inside an evacuated tube, further reducing convective and conductive heat losses. These collectors can reach higher output temperatures, 150 °C and even 200 °C are possible.
Modelling and optimisation
The goal of the research is to improve the efficiency and economic viability of solar-assisted heating systems and to assess their potential for different regions and applications. Thermo-economic models of collectors and heating systems are used to investigate the performance of different configurations and to optimise system design and operation. Market research is used to identify the range of collectors available on the market and to obtain ranges for performance and costs. Solar-thermal collectors are also implemented in whole-energy system models to assess their system value and to compare them to other conventional and low-carbon heating systems.
Selected publications:
[1] Unamba CK, Sapin P, Li X, Song J, Wang K, Shu G, Tian H, Markides CN. Operational optimisation of a non-recuperative 1-kWe organic rankine cycle engine prototype. Applied Sciences 2019; 9:3024. https://doi.org/10.3390/app9153024
[2] Freeman J, Hellgardt K, Markides CN. Working fluid selection and electrical performance optimisation of a domestic solar-ORC combined heat and power system for year-round operation in the UK. Applied Energy 2017; 186:291-303. https://doi.org/10.1016/j.apenergy.2016.04.041
[3] Freeman J, Guarracino I, Kalogirou SA, Markides CN. A small-scale solar organic Rankine cycle combined heat and power system with integrated thermal energy storage. Applied Thermal Engineering 2017; 127:1543-54. https://doi.org/10.1016/j.applthermaleng.2017.07.163
[4] Freeman J, Hellgardt K, Markides CN. An assessment of solar-powered organic Rankine cycle systems for combined heating and power in UK domestic applications. Applied Energy 2015; 138:605-20. https://doi.org/10.1016/j.apenergy.2014.10.035
[5] Markides CN. Low-concentration solar-power systems based on organic Rankine cycles for distributed-scale applications: Overview and further developments. Frontiers in Energy Research 2015; 3:47. https://doi.org/10.3389/fenrg.2015.00047
3. High-performance hybrid photovoltaic-thermal (PVT) solar collectors and systems
Photovoltaic-thermal (PVT) technology has been attracting increased interest as a solution that promotes broader solar utilisation. In PVT collectors, heat is removed from the PV cells by attaching a thermal absorber to the bottom the cells, thus simultaneously producing electricity and useful thermal energy from the same aperture area with a total efficiency (electrical plus thermal) in excess of 70%. The thermal output is usually limited to 60-80 °C, which can be used for domestic hot water or air heating for households or commercial use. A challenge is to identify PVT collector designs that can generate high-temperature thermal energy while not sacrificing the PV electrical efficiency. In order to unlock a wider range of applications driven by higher-temperature thermal energy (such as solar cooling and power generation), the CEP laboratory is currently working on high-performance PVT solar collectors and systems.
3.1. High-temperature high-efficiency PVT collectors
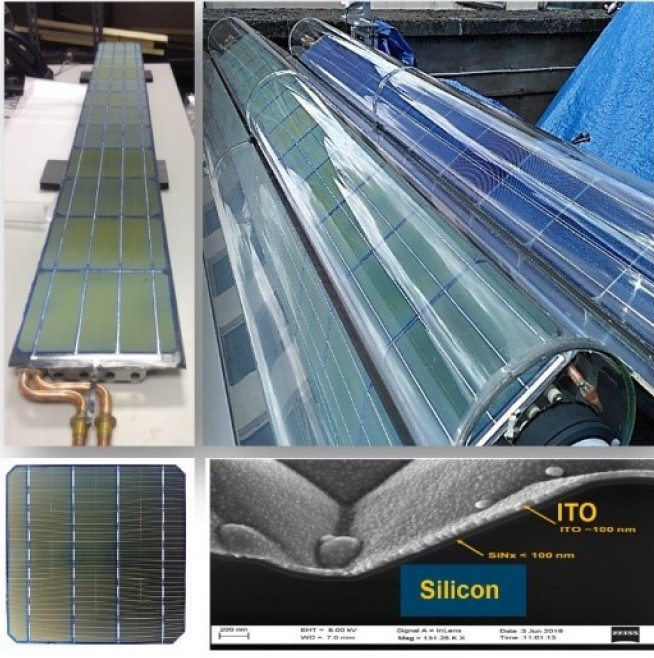
Advances in PV materials, energy/flow management, innovative loss-suppression solutions are required to enable disruptive improvements in the performance of PVT collectors. The CEP laboratory has successfully demonstrated that high electrical and thermal performance at high temperatures are attainable by PVT collectors featuring advanced heat-suppression designs, including vacuum insulation, low-emissivity coatings, and low-temperature-coefficient PV cells. The most advanced collector design is projected to have double the thermal efficiency compared to present commercially available PVT collectors, and to provide 1.5 and 2 times the revenue or carbon savings of separate PV modules and solar-thermal collectors, respectively. Research in the CEP Energy Division is centred in the modelling, design, fabrication and testing of the proposed PVT collector as part of a wider solar-energy-based heat, power and cooling (solar-based tri-generation) system.
3.2. Next-generation spectral-splitting PVT (SS-PVT) collectors
Spectral-splitting hybrid photovoltaic-thermal (SS-PVT) collector technology has emerged recently as a promising route toward next-generation high-performance solar harvesting, i.e., splitting the incident sunlight into separate bands, one that is well-suited to conversion into electricity, which is directed to the PV cells, and a second one that is absorbed as high-temperature thermal energy. Members of the CEP laboratory have developed comprehensive numerical and theoretical models, and use them to design, characterise and optimise such next-generation high-performance SS-PVT collectors. Work also focuses on developing advanced spectral-splitting optical materials (nanofluids and nanocomposite etc.). Several prototypes have been built and tested under a solar simulator as well as real outdoor environment. A recent Imperial College spin-out company, Solar Flow, has been founded to develop and commercialise such next-generation hybrid solar technologies. Recent research by the CEP laboratory was featured in Imperial College London News (link) and the PV Magazine (link) in 2021.

3.3. Solar PVT energy systems
Hybrid PVT collectors have both electrical and thermal outputs, which makes them highly suitable for meeting the complete energy needs of end-users, also with excellent flexibility for integrating with other technologies (refrigerators, heat pumps, power generation units, energy storage units, etc.). The tools and knowledge gained from component-level characterisation and system-level integration in the CEP laboratory are being used to assess and understand the technoeconomic potential of such “all-in-one” solar energy systems, and to identify appropriate designs and optimal operation strategies for specific applications of interest, such as for meeting the energy demands of domestic buildings, sport centres, university campuses, dairy farms, greenhouses, etc.
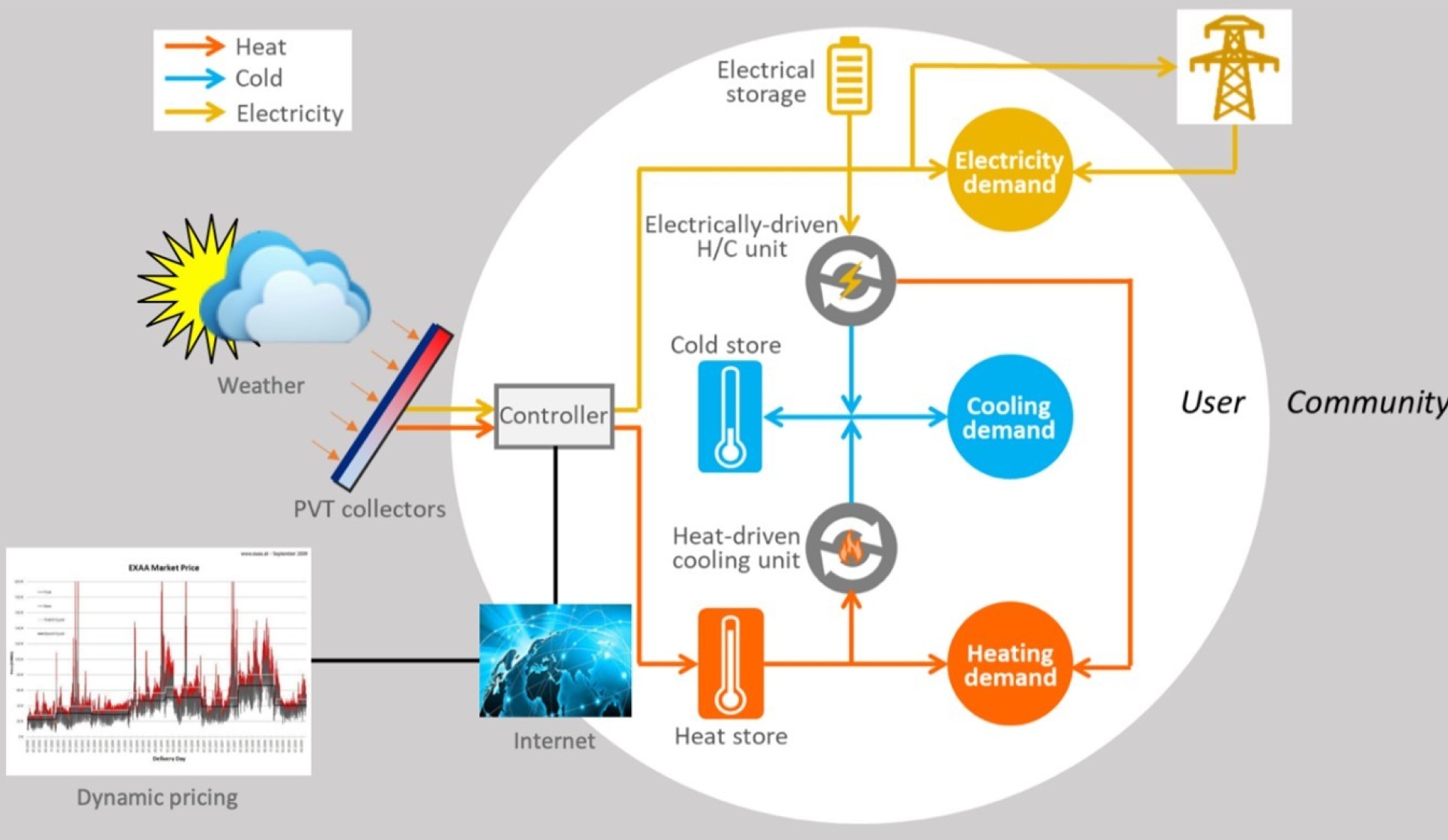
Selected publications:
[1] Huang G, Markides CN. Spectral-splitting hybrid PV-thermal (PV-T) solar collectors employing semi-transparent solar cells as optical filters. Energy Conversion and Management 2021; 248: 114776. https://doi.org/10.1016/j.enconman.2021.114776
[2] Huang G, Wang K, Markides CN. Efficiency limits of concentrating spectral-splitting hybrid photovoltaic-thermal (PV-T) solar collectors and systems. Light: Science & Applications 2021; 10: 28. https://doi.org/10.1038/s41377-021-00465-1
[3] Mellor A, Alvarez DA, Guarracino I, Ramos A, Lacasta AR, Llin LF, Murrel AJ, Paul DJ, Chemisana D, Markides CN, Ekins-Daukes NJ. Roadmap for the next-generation of hybrid photovoltaic-thermal solar energy collectors. Solar Energy 2018; 174, 386-398. https://doi.org/10.1016/j.solener.2018.09.004
[4] Herrando M, Markides CN, Hellgardt K. A UK-based assessment of hybrid PV and solar-thermal systems for domestic heating and power: System performance. Applied Energy 2014; 122: 288-309. https://doi.org/10.1016/j.apenergy.2014.01.061
4. Low-carbon heating technologies
One of the research activities of the group involves the design of low-carbon technologies specifically targeted for heat decarbonisation. This involves the development of holistic, multiscale modelling frameworks, within which alternative heat provision solutions are compared and simultaneously optimised (design, operation) in an effort to find the best, sustainable technology mix to replace the widely used gas boilers. Focus is placed on the detailed modelling of relevant technologies in order to capture the complex characteristics and trade-offs between underlying components and materials of the possible solutions within whole-energy system models, thereby providing insights not only to policy makers and system operators, but also to technology manufacturers and installers, along with innovative, optimal designs from a whole-system perspective. This research is a core element of EPSRC Programme Grant ‘Integrated Development of Low-Carbon Energy Systems’ (IDLES), within which we collaborate with research groups across a number of Departments at Imperial (Electrical Engineering, Business School, CEP, etc.) as well as external partners (EDF, ABB, IBM, etc.).
Recent progress in this field includes the development of comprehensive technoeconomic models of single-stage-compressor low-temperature heat pumps (air-source, ground-source), which combine dedicated heat-exchanger models, compressor efficiency maps and suitable equipment costing techniques to assess various designs with alternative, ‘green’ working fluids. These are being utilised to provide reliable off-design performance characteristics to whole-energy capacity-expansion and unit-dispatch models focusing on the electrification of heat, assessing the implications on the generation mix of the future energy network. Investment and operation decisions at the end-use level can significantly contribute to attenuating impacts of electrification on the power grid.
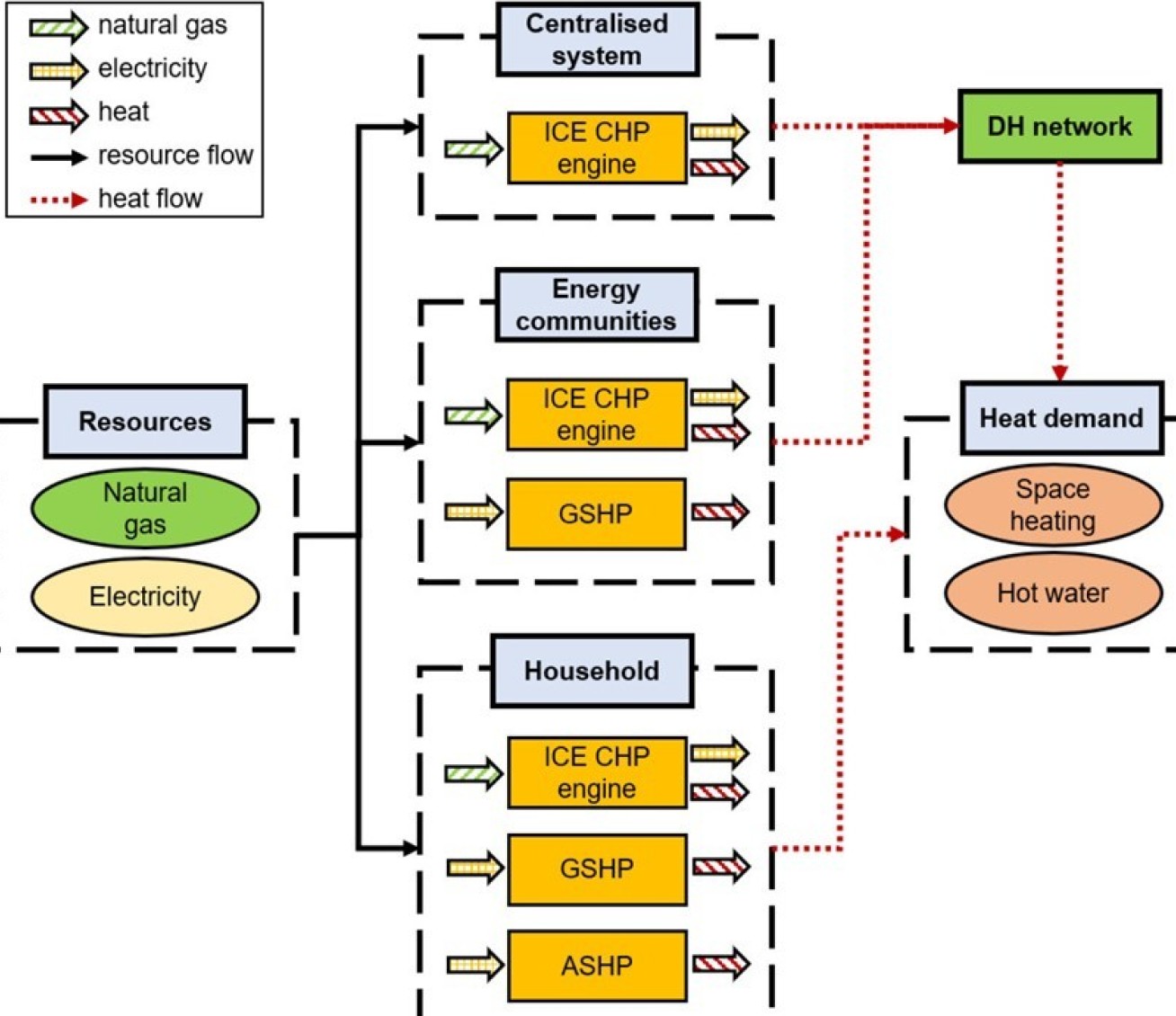
Cost and performance data for relevant heating technologies (heat pumps, cogeneration systems, boilers etc.,) were used to perform a comprehensive comparison of combined heat and power (CHP) systems and heat pumps in centralised and distributed heating systems. The novelty of the work lies in the use the thermo-economic models (data-driven, thermodynamic, sizing/costing) to capture realistic technology characteristics (scale effects and off-design performance) and therefore provide an informed comparison of the possible technology and design/sizing options from a whole-energy system’s perspective. Uncertainty propagation analysis is also conducted to quantify the uncertainty on the final results with multi-fidelity approaches and demonstrate the necessity of using refined comprehensive design models to reliably capture the full potential of considered technologies.
Furthermore, members of the CEP Energy Division are developing a library that consists of extensive price and performance data of commercially available technologies for low-carbon energy systems on the UK market, including domestic and commercial applications. The library contains useful information for energy-system and technology modellers in their efforts to capture the techno-economic characteristics of different technology options, minimise uncertainties and suggest reliable system- and technology-design strategies. The library is open-access and can be found here.
Selected publications:
[1] Olympios AV, Mersch M, Sapin P, Pantaleo AM, Markides CN. Library of price and performance data of domestic and commercial technologies for low-carbon energy systems (Version 1) [Data set]. Zenodo. 2021. http://doi.org/10.5281/zenodo.4692649
[2] Mersch M, Olympios AV, Sapin P, Mac Dowell N, Markides CN. Solar-thermal heating potential in the UK: A technoeconomic whole-energy system analysis. The 34rth International Conference on Efficiency, Cost, Optimization, Simulation and Environmental Impact of Energy Systems, Sicily, Italy, 2021.
[3] Olympios AV, Pantaleo AM, Sapin P, Markides CN. On the value of combined heat and power (CHP) systems and heat pumps in centralised and distributed heating systems: Lessons from multi-fidelity modelling approaches. Applied Energy 2020; 274: 115261. https://doi.org/10.1016/j.apenergy.2020.115261
[4] Sapin P, Simpson M, Olympios AV, Mersch M, Markides CN. Cost-benefit analysis of reversible reciprocating-piston engines with adjustable volume ratio in pumped thermal electricity storage. The 33rd International Conference on Efficiency, Cost, Optimization, Simulation and Environmental Impact of Energy Systems, Osaka, Japan, 2020. http://hdl.handle.net/10044/1/80837
[5] Olympios AV, Hoisenpoori P, Mersch M, Pantaleo A, Simpson M, Sapin P, Mac Dowell N, Markides CN. Optimal design of low-temperature heat-pumping technologies and implications to the whole energy system. The 33rd International Conference on Efficiency, Cost, Optimization, Simulation and Environmental Impact of Energy Systems, Osaka, Japan, 2020. http://hdl.handle.net/10044/1/80718
5. Large-scale electricity storage
The CEP Laboratory has also been active in thermo-economic analyses of large-scale electricity storage systems at the scale of multiple MW/MWh. More specifically, thermo-mechanical energy storage systems are investigated, which are potentially cheaper, more robust alternatives to grid-scale batteries. Technologies of interest include compressed-air energy storage (CAES), liquid-air energy storage (LAES) and pumped-thermal electricity storage (PTES). These technologies have the ability to utilise low-carbon, low-cost electricity during off-peak demand periods for charging. The electricity is then released when demand is high. This can help reduce curtailing of renewable, increase the share of low-carbon electricity generation and reduce the need for backup generation capacities. The technical and economic characteristics of these systems are such that, especially at high discharge power ratings and long discharge durations, they can offer promising performance, along with high lifetimes, low specific costs, low ecological footprints, and unique sector-coupling features.
Thermodynamic models of these thermo-mechanical energy storage systems are used for analyses of their technical performance and the identification of influential parameters. Economic assessments are undertaken based on economic models which also account for the thermodynamic characteristics associated with each technology for its charging, storage and discharging elements. Economic evaluations consider different cost components such as initial capital expenditure, energy costs, and operational and maintenance costs. Also, financial indicators such as power capital costs (i.e. capital cost divided by power capacity), energy capital costs (i.e. capital cost divided by the energy capacity), levelised cost of storage, and required sell-to-buy electricity prices are studied and compared for the different systems. These thermo-economic models are finally used to optimise systems, components and their operation, and to assess and compare the performance of different technologies.
The economic competitiveness of newly proposed technologies can be challenging due to the lack of cost data or case studies. The combination of thermodynamic and economic analyses through a unified thermo-economic framework can give estimates of the technical and economic competitiveness of these systems under different scenarios as well as in comparison to other electricity storage technologies.
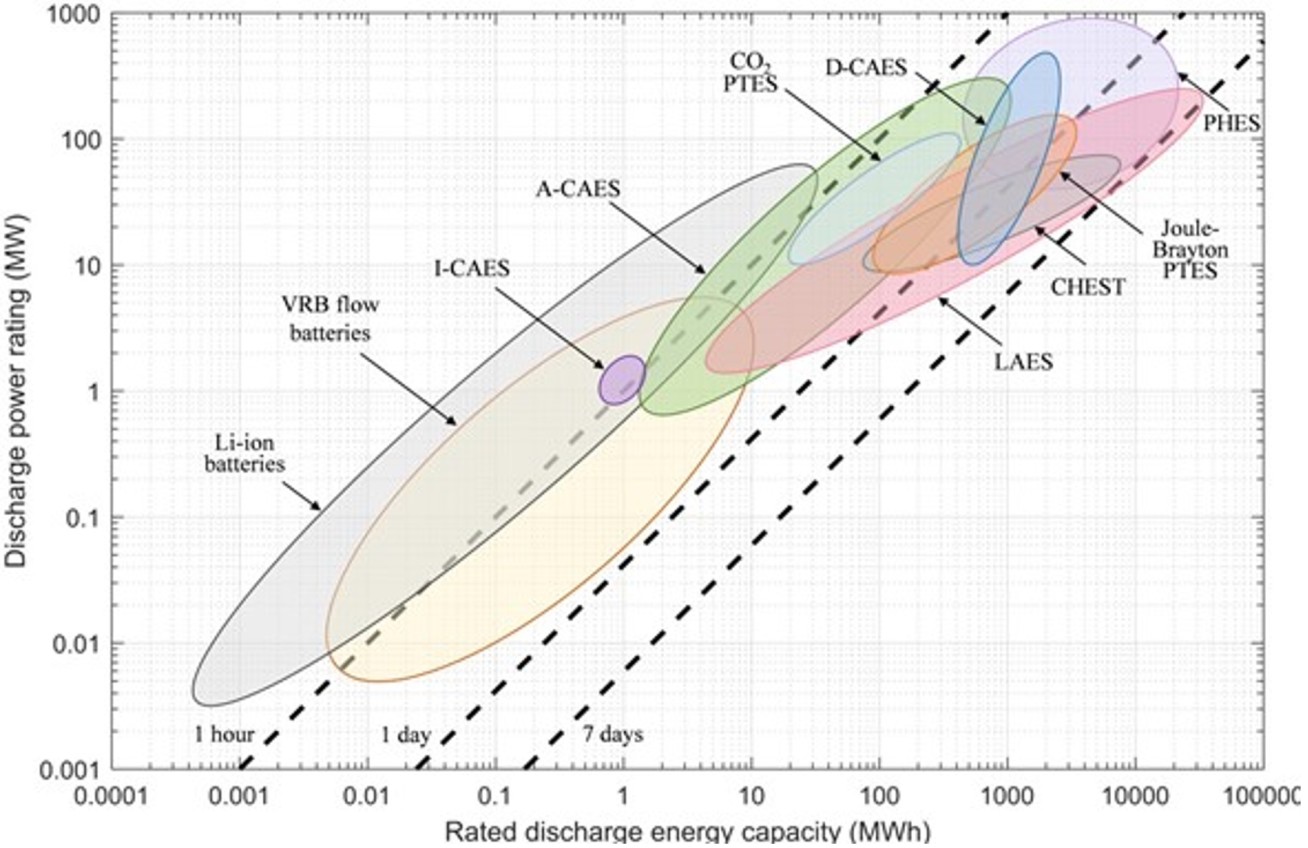
Selected publications:
[1] Zhao Y, Song J, Liu M, Zhao Y, Olympios AV, Sapin P, Yan J, Markides CN. Thermo-economic assessments of pumped-thermal electricity storage systems employing sensible heat storage materials. Renewable Energy 2022; 186: 431-56. https://doi.org/10.1016/j.renene.2022.01.017
[2] Olympios AV, McTigue JD, Farres-Antunez P, Tafone A, Romagnoli A, Li Y, Ding Y, Steinmann WD, Wang L, Chen H, Markides CN. Progress and prospects of thermo-mechanical energy storage – A critical review. Progress in Energy 2021; 3: 022001. doi.org/10.1088/2516-1083/abdbba
[3] Zhao Y, Liu M, Song J, Wang C, Yan J, Markides CN. Advanced exergy analysis of a Joule-Brayton pumped thermal electricity storage system with liquid-phase storage; Energy Conversion and Management 2021; 231: 113867. https://doi.org/10.1016/j.enconman.2021.113867
[4] Georgiou S, Aunedi M, Strbac G, Markides CN. On the value of liquid-air and pumped-thermal electricity storage systems in low-carbon electricity systems. Energy 2019; 193: 11660. doi.org/10.1016/j.energy.2019.116680
[5] Georgiou S, Shah N, Markides CN. A thermo-economic analysis and comparison of pumped-thermal and liquid-air electricity storage systems. Applied Energy 2018; 216: 1119-33. doi.org/10.1016/j.apenergy.2018.04.128
6. Thermal energy storage
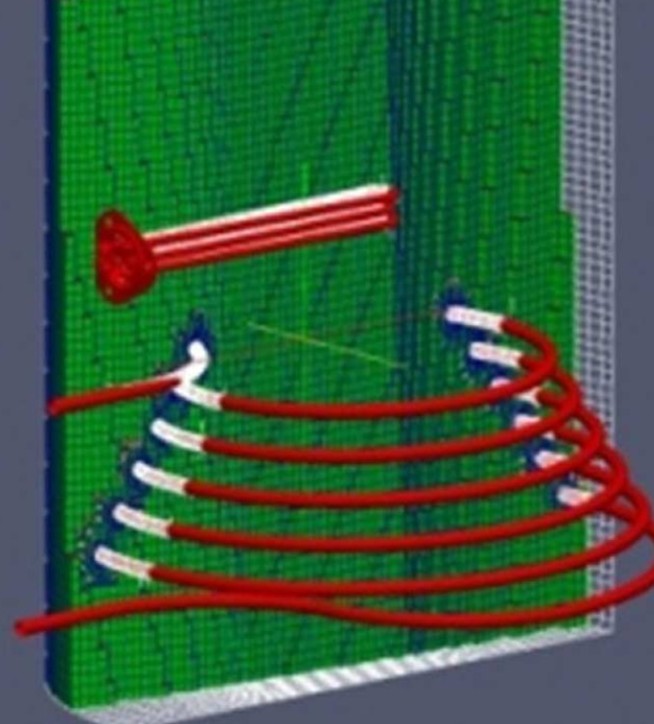
The desire to utilise energy efficiently has led recently to an increased interest in thermal energy storage (TES) systems. TES can play a vital role in future energy systems, by enabling a reduction in the discordance (in time or rate) between the production or availability of energy and its consumption. By storing thermal energy locally, for later use when this is required (i.e. for space heating, or hot water), the peak energy load can be reduced and a lower-cost, off-peak energy input utilised instead.
The integration of latent heat storage solutions into modern heating and cooling systems has the potential to enhance the overall system performance compared to standard hot-water systems (radiators and tanks) due to an augmentation of the stored heat by the latent heat of a suitable material.
Aiming to characterise the dynamic behaviour and performance of an active thermal storage system for domestic applications, CEP has undertaken an investigation involving computational predictions complemented by experimental measurements in order to capture the time-varying interplay between fluid flow and heat transfer in a system based on the use of a hydrated salt Phase Change Material (PCM).
Computational modelling makes use of CFD toolbox OpenFOAM®. The CEP laboratory is also equipped with facilities for the experimental study of TES systems.
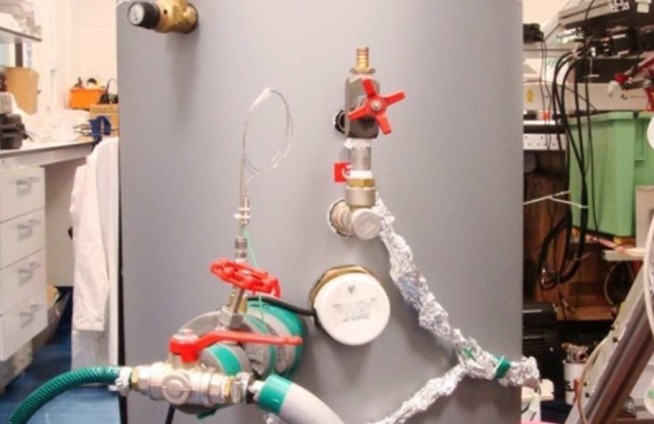
Highlights
- Members of the CEP laboratory developed an open-access library consisting of extensive price and performance data of commercially available technologies for low-carbon energy systems on the UK market, including domestic and commercial applications. Download the library here.
- Dr. Huang et al. et al published a new study ‘Efficiency limits of concentrating spectral-splitting hybrid photovoltaic-thermal (PV-T) solar collectors and systems’ in Light: Science & Applications in 2021. Download the article here.
- Olympios et al. published a review paper: "Progress and prospects of thermo-mechanical energy storage—a critical review' in Progress in Energy in 2021. Download the aritcle here.
- Dr. Sapin published a research highlight blog explaining how Project 2 of the Integrated Development of Low-carbon Energy Systems (IDLES) programme is building a collection of comprehensive thermodynamic and costing models for energy technologies to determine the role they might play in an integrated future energy system.
- Dr. Ing. Constanze Bongs and Axel Oliva from Fraunhofer ISE, Group Building Systems Technology visited the CEP group on 8 May 2017 and gave the Seminar “The German Fraunhofer Society and it´s pathway to energy efficient buildings “.
- Dr Alba Ramos et al published a briefing paper with the Grantham Institute: 'Solar-Thermal and Hybrid Photovoltaic-Thermal Systems for Renewable Heating', Grantham Institute Briefing paper No 22, 2017. DOI: 10.13140/RG.2.2.10473.29280. Download the article here.
- The paper “A Framework for the Analysis of Thermal Losses in Reciprocating Compressors and Expanders” by Richard Mathie, Christos N. Markides and Alexander J. White is now the 8th all-time most downloaded paper published in journal Heat Transfer Engineering.
-
On 12 October 2016, Michel De Paepe Professor of Thermodynamics & Heat Transfer in the Department of Flow, Heat and Combustion Mechanics of the Faculty of Engineering and Architecture at Ghent University visited the CEP group and gave a semirar "Waste heat recovery by ORC technology: How to make it happen".