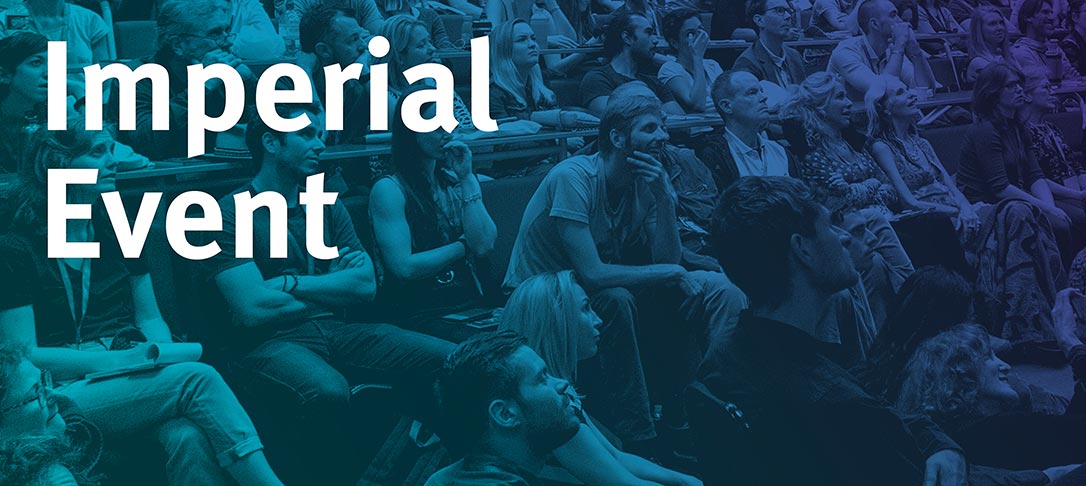
*We have now reached capacity and are unable to accept any further registrations.*
20 May schedule:
09.45 Welcome – Aron Walsh
10:00 Sandrine Heutz (Imperial College London) Spin coupling in molecular thin films: opportunities from solar to quantum
10:30 Matthew Cliffe (University of Nottingham) Modular magnetism: 2D coordination frameworks
Tea & Coffee
11:30 David Scanlon (University of Birmingham) Computational prediction and experimental realisation of new transparent conducting oxides
12:00 Nuria Tapia-Ruiz (Imperial College London) Building and understanding the behaviour of high-performance batteries by rationalising structural electrode design
12:30 Flash Poster Presentations
Lunch sponsored by RSC journals Materials Horizons and Nanoscale Horizons & Posters
14:00 Amita Ummadisingu (University College London) Multi-length scale structure of two-dimensional halide perovskites
14:30 Saiful Islam (University of Oxford) From batteries to solar cells: exploring energy materials on the atomic scale
15:00 Shelly Conroy (Imperial College London) Even hard materials are soft at the nanoscale
Tea & Coffee
16:00 Romain Gautier (CNRS Institute des Materiaux de Nantes Jean Rouxel) Predicting the synthesis of new low-dimensional hybrid perovskite by machine learning
16:30 Oliver Fenwick (Queen Mary University London) Thermoelectric properties of halide perovskites
17:00 Closing – James Durrant
Day 2, 21 May is focused on early career researchers.
Session 1 (Blackett Laboratory, LT2). Chair: Dr Filip Podjaski
1000 Welcome: Dr Filip Podjaski, Dr Julianna Panidi
1005 Invited internal speaker: Dr Heather Au, Imperial College London
Carbon fibre hosts for sustainable lithium-sulfur battery electrodes
1030 Gong Yi, Imperial College London
Ampere-hour-scale soft-package potassium ion hybrid capacitors enabling 6-minute fast charges
1045 Dr Seung-Jae Shin, Imperial College London
Atomically resolved electrochemical interface via computational chemistry
1100 Dr Linnea Lind, Imperial College London
Fe-complexes for solar energy conversion
1115 Tea/coffee and posters (Foyer level 2/3)
Session 2 (Blackett Laboratory, LT2)
1140 Invited external speaker: Dr Miguel Anaya, University of Cambridge
Photonics for enhanced perovskite optoelectronic devices
1205 Ziyuan Ge, Imperial College London
Investigating the electron phonon coupling of 2D Sn perovskites excited states using impulsive Raman spectroscopy
1220 Irea Mosquera Lois, Imperial College London
Machine learning point defect reconstructions
1235 Davide Nodari, Imperial College London
Dark current in broadband perovskite-organic heterojunction photodetectors controlled by interfacial energy band offset
1250 George Creasey, Imperial College London
Design and development of photoelectrochemical reactors for solar H2 production
1305 Lunch and posters (Foyer level 2/3) Sponsored by RSC journals Materials Horizons and Nanoscale Horizons
Session 3 (Blackett Laboratory, LT2)
1345 Invited external speaker: Dr Chris Proctor, University of Oxford
Mulitmodal bioelectronics
1410 Ming Xu, Imperial College London
Minimally invasive power supply with long-term stability for implantable electronics
1425 Berjaeu Officer, Imperial College London
Rational design of robust mesh bioelectronics for stable physiological interrogation of cardiomyocytes
1440 Tea/coffee and posters (Foyer level 2/3)
Session 4 (Blackett Laboratory, LT2)
1510 Invited external speaker: Ian Jacobs, University of Cambridge
In-operando spectroscopic characterization of non-equilibrium states in a highly doped polymer
1535 Dr Martina Rimmele, Imperial College London
Soluble conjugated polymers without side chains: macrocycles as comonomers
1550 Jack Coker, Imperial College London
Perpendicular crossing chains enable high mobility in a non-crystalline conjugated polymer
1605 Charlie Henderson, Imperial College London
Charge transfer complex formation between organic interlayers drives light-soaking in large area perovskite solar cells
1620 Dr Matyas Daboczi, Imperial College London
Perovskite and organic photoanodes for days-long stable solar water splitting with photocurrents close to the theoretical limit
1635 Closing
If you are interested in presenting a poster, please contact Lisa Bushby. The lunch break is very kindly sponsored by RSC journals Nanoscale Horizons and Materials Horizons.
£100 poster prize sponsored by RSC Applied Interfaces
£100 poster prize sponsored by Journal of Materials Chemistry C
£100 poster prize sponsored by Sustainable Energy & Fuels
_______________________________________________________
ABSTRACTS AND BIOGRAPHIES
Miguel Anaya, University of Cambridge
Photonics for enhanced perovskite optoelectronic devices
BIO
Miguel Anaya is a Royal Academy of Engineering Research Fellow at the Department of Chemical Engineering and Biotechnology, and a Research Fellow at Darwin College, University of Cambridge. He graduated from Autonomous University of Madrid in 2012 with a B.S.+M.S. in Physics (First Class), and he obtained a second M.S. in Materials Science in 2013 at the University of Seville (First Class Honours). He completed his PhD at the Spanish National Research Council in 2018, with recognition from the Spanish Royal Society of Physics as the best Thesis in Experimental Physics.
Miguel leads a team focussed on the modelling, fabrication and characterisation of perovskite-based devices at the Optoelectronic Materials and Device Spectroscopy Group. For his work, he has received the SPIE Scholarship, the E-MRS Student Award, Best Young Investigator 2016 by the City Hall of Seville and has been distinguished as Emerging Investigator 2021 by the Royal Society of Chemistry. Despite his early career stage, to date Miguel has raised ca. £1.5M.
Heather Au, Imperial College London
Carbon fibre hosts for sustainable lithium-sulfur battery electrodes
BIO
Heather is a Royal Society University Research Fellow in the Department of Chemical Engineering at Imperial College London. She obtained her PhD in 2017 from Imperial College developing covalent modification strategies on carbon nanomaterials. She was a postdoctoral research associate at Queen Mary University of London and Imperial College, where her research interests shifted to investigating charge storage mechanisms in sodium-ion battery anodes, and later a Faraday Institution Research Fellow, working on the development of engineered carbon hosts for sulfur cathodes in lithium-sulfur batteries. Heather was awarded a Royal Society University Research Fellowship in 2023, allowing her to establish an independent research team exploring sustainable materials for structural energy storage.
Matthew Cliffe, University of Nottingham
Modular magnetism in 2D coordination frameworks
Coordination framework materials, where metal ions are connected by molecular ligands into extended networks, are modular: their components can be exchanged while retaining the overall structural topology. This modularity allows for design strategies that are challenging to realise in conventional atomic inorganic materials. In this talk I will discuss recent work from the Cliffe Group exploring the magnetism of layered coordination frameworks, demonstrating how chemical control of framework structure can produce varied function from non-collinear spin textures to routes towards quantum phases. I will also show how neutron scattering can provide otherwise challenging to obtain insights into both the ground states and interactions of magnetic materials. I will highlight in particular our work on metal thiocyanates,[1,2,3,4] and layered dense metal organic frameworks.[5]
[1] M. J. Cliffe et al., Phys Rev B, 97, 144421 (2018)
[2] E. N. Bassey et al., Inorg Chem, 59, 11627 (2020)
[3] M. Geers et al., Chem. Sci.14, 3531 (2023)
[4] M. Geers et al., Phys. Rev. B 108, 144439 (2023)
[5] J. Pitcairn et al., J. Am. Chem. Soc., 145, 1783(2023)
BIO
Matthew Cliffe is an Associate Professor at the School of Chemistry at the University of Nottingham. He obtained his DPhil from the University of Oxford and was a Junior Research Fellow at Sidney Sussex College, University of Cambridge. He joined the University of Nottingham in 2018 as the inaugural Hobday Fellow. He was awarded a UKRI Frontiers (ERC Starting Grant Guarantee) grant in 2022. His research focusses on how we can control and characterise novel coordination frameworks to generate new and unusual magnetic and electronic behaviour.
George Creasey, Imperial College London
Design and Development of Photoelectrochemical Reactors for Solar H2 Production
G. H. Creasey1,2, A. W. Moelich3, J. W. Rodriguez Acosta1, T. Shalvey4, D. A. Garcia-Osorio4, T. McCallum2, L. O’Neil1, G. Ai2, J. Major5, A. Cowan4, C. McGregor3, A. Kafizas2, A. Hankin1
1 Imperial College London Department of Chemical Engineering, London, SW7 2AZ, United Kingdom
2 Imperial College London Department of Chemistry, London, W12 0BZ, United Kingdom
3 Stellenbosch University Department of Mechanical and Mechatronic Engineering, Stellenbosch, 7602, South Africa
4 University of Liverpool Department of Chemistry, Liverpool, L69 7ZD, United Kingdom
5 University of Liverpool Department of Physics, Liverpool, L69 7ZE, United Kingdom
Industrial deployment of photoelectrochemical systems for hydrogen production via solar-powered water splitting still necessitates considerable progress to be made in the development and operation of scalable demonstration units. Research efforts are presently largely focused on the development of photoelectrode materials that perform the combined function of light absorption, charge transport and reaction catalysis, at the same time as demonstrating adequate chemical stability and requiring low production costs. However, in addition to material development, the engineering of scalable photoelectrochemical reactors that would utilise these materials is also constrained by many requirements that must be satisfied simultaneously. The factors that govern reactor design include: (i) electrode scale-up; (ii) mode of photo-electrode illumination; (iii) current distribution (and associated losses); (iv) the utilisation of a membrane; (v) electrolyte recirculation; (vi) product harvesting; (vii) heat dissipation.[1] These and other design considerations must enable the reactor to be suitable for operation in industrial conditions.
We have developed a photoelectrochemical demonstration unit capable of splitting water into hydrogen and oxygen using only photon energies. The reactor was irradiated using natural sunlight in March at Stellenbosch University (33.93° S, 18.86° E), while mounted on a 2-axis tracking platform. Light was directed laterally both into the (photo)cathode and photoanode compartments, which were separated by an ion-permeable membrane; this was achieved via two methods that were compared with each other: (i) using mirrors (Ag-coated mirror for the (photo)cathode side and Al-coated mirror for the photoanode side) and (ii) linear Fresnel lenses coupled with stepped Al waveguides. The latter irradiation method delivered concentrated light, with a concentration factor of up to 15.
The reactor was operated in two modes:
Photoelectrochemical (PEC), utilising an FTO|WO3|BiVO4|NiFeOx photoanode and a FTO|Au|Sb2Se3|CdS|TiO2|Pt photocathode[2];
PV-assisted photoelectrochemical (PV-PEC), utilizing the same FTO|WO3|BiVO4|NiFeOx photoanode, Ni cathode and an externally mounted c-Si PV.
I shall discuss the experimental results from reactor testing, the performance under various modes of irradiation, and the effects of electrode materials, geometries and relative configurations within the reactor on its design, overall performance and further scale-up, as well as the future role of photoelectrochemical systems in energy storage.
[1] A review of inorganic photoelectrode developments and reactor scale-up challenges for solar hydrogen production, Adv. Energ. Mater., 2021, 11, 2003286.
[2] Benchmark performance of low-cost Sb2Se3 photocathodes for unassisted solar overall water splitting, Nat. Commun., 2020, 11, 861.
Matyas Daboczi, Imperial College London
Perovskite and organic photoanodes for days-long stable solar water splitting with photocurrents close to the theoretical limit
Matyas Daboczi1, Flurin Eisner2, Joel Luke2, Junyi Cui1, Filipp Temerov1,3, Shi Wei Yuan2, Noof Al Lawati1, Jolanda Simone Müller2, Ji-Seon Kim3, Jenny Nelson2, Salvador Eslava1
1 Department of Chemical Engineering and Centre for Processable Electronics, Imperial College London, London SW7 2AZ, UK
2 Department of Physics and Centre for Processable Electronics, Imperial College London, London SW7 2AZ, UK
3 Nano and Molecular Systems Research Unit, University of Oulu, Oulu, FI-90014 Finland
Conversion of solar energy into hydrogen by photoelectrochemical water splitting is an emerging technology with large potential for sustainable green hydrogen generation. However, reaching the combination of high performance, stable and inexpensive photoelectrodes remains a scientific challenge. Solution-processable perovskite and organic photoactive materials have shown remarkable efficiency in solar cells, and they are extremely promising candidates for photoelectrochemical devices, but their application so far has been limited by their instability in aqueous media. In this presentation, we will show our most recent results on perovskite and organic photoanodes applying NiFeOOH-functionalized self-adhesive graphite sheet, providing a simple, cost-effective approach to prevent degradation by the aqueous environment and eliminate almost all electrical losses between the photoactive layer and the water oxidation-catalyst.
The all-inorganic perovskite photoelectrodes incorporate solely Earth-abundant materials and apply a low annealing temperature carbon paste with tuned energy level CsPbBr3 photoactive layer. Controlling the perovskite phase by a facile chemical bath method allows to achieve a pure 3D CsPbBr3 layer with micrometre-size grains and increased electric field at the electron transport layer / perovskite interface helping separation and transfer of photogenerated charge-carriers. These enhancements combined with the electrocatalyst-functionalized graphite sheet led to CsPbBr3 photoelectrodes with 8.1 mA cm-2 photocurrent density at +1.23 VRHE (close to the radiative efficiency limit of CsPbBr3) with record perovskite photoanode stability: 100% of stabilized photocurrent density maintained for more than 100 h. Devices with >1 cm2 area, and low-temperature processing will also be demonstrated.1
Organic photoanodes containing polymer:non-fullerene acceptor absorber layer (PM6:D18:L8-BO) achieve breakthrough photocurrent densities over 25 mA cm‑2 at +1.23 VRHE and remarkable, days-long operational stability. We will discuss strategies of how the continuous operation of the organic photoanode could be extended even further. Finally, polymer:polymer photoelectrodes, as well as monolithic organic tandem photoanodes (i.e., two photoactive layers integrated into one photoanode to generate high photovoltage) with exceptionally low, negative onset potential and bias-free water splitting reaching up to 5% solar-to-hydrogen efficiency will be presented.
Daboczi, M. et al. Scalable All-Inorganic Halide Perovskite Photoanodes with >100 h Operational Stability Containing Earth-Abundant Materials. Advanced Materials 2304350 (2023) doi:10.1002/ADMA.202304350.
Romain Gautier, Centre National de la Recherche Scientifique (CNRS)
Predicting the synthesis of new low-dimensional hybrid perovskite by machine learning
The discovery of new materials with specific properties is a complex task due to the drastic effects that very slight modifications on the synthesis of materials can have on the crystal structures and the resulting properties. Machine learning can be a very useful tool in such situations and can drastically accelerate the discovery of new functional materials with specific structure type. In this presentation, the accelerated discovery of new hybrid perovskites by machine learning will be presented. These materials have recently shown a great potential in optoelectronic applications. For this reason, many research groups are currently exploring the chemical system of hybrid metal halides to discover new low dimensional hybrid perovskites. However, discovering such materials is challenging as the necessary structure determination by X-ray diffraction is time consuming and non-perovskite compounds are very often synthesized instead of the more interesting perovskites. In this presentation, two approaches will be introduced which both guide us towards the synthesis of new hybrid perovskites: (i) the use of deep learning to automatically identify hybrid perovskites from powder X-ray diffraction patterns,[1] and (ii) the use of molecular descriptors to identify organic amines with high probability to lead to the synthesis of hybrid perovskites.[2] From this machine learning tools, the scientists’ ability to predict the synthesis of new hybrid perovskites is largely augmented and such algorithms could be included in autonomous materials discovery cycles in the future.
[1] F. Massuyeau, T. Broux, F. Coulet, A. Demessence, A. Mesbah, R. Gautier, Advanced Materials 2022, 34, 2203879
[2] R. Laref, F. Massuyeau, R. Gautier, Small 2023, 2306481
BIO
Romain Gautier is a research scientist at the Centre National de la Recherche Scientifique (CNRS) in the Institut des Matériaux de Nantes Jean Rouxel. He obtained his Ph.D. degree from the Ecole Nationale Supérieure de Chimie de Rennes in inorganic and materials chemistry in 2010. He is the recipient of the CNRS Bronze medal and the National Chinese Award “1000 Young Talents”. His current research interests include the design, synthesis, and characterization of new inorganic and hybrid materials with properties of second harmonic generation and luminescence. He has also been utilizing machine learning techniques to accelerate the discovery of new materials with specific structures and properties.
Ziyuan Ge, Imperial College London
Investigate the electron phonon coupling of the 2D Sn perovskites excitonic excited states using the impulsive Raman spectroscopy
Ziyuan Ge1, Meihuizi Jiang1, Nimrod Benshalom2, Guy Reuveni2, Haoqing Ning1, Tom Hopper1,3, Tong Wang1, Omer Yaffe2, Artem Bakulin1
1Department of Chemistry and Centre for Processable Electronics, Imperial College London, W12 0BZ, United Kingdom
2Department of Chemical and biological physics, Weizmann institute of Science, 76100, Israel
3Department of Energy, Stanford University, 94025, California, USA
Author e-mail address: z.ge20@imperial.ac.uk
2D perovskite known to have superior luminescence properties is sought to be exploited for their novel uses, e.g., lasing, thermoelectric, etc…1,2 Studying electron-phonon coupling is vital for understanding the dynamics of carriers, which in turn is key to unravelling the unique optical and electronic properties of the photovoltaic material.3 Meanwhile, the determination of the phonon modes coupling to the electronic states has always been a challenge. In this experiment, we have observed a strong coherent vibrational signal of (PEA)2SnI4 perovskite at room temperature using the impulsive Raman. We successfully determine the modes, 33cm-1and 22cm-1, that has strong coupling to the excitonic excited states.
1 A. L. Alvarado-Leaños, D. Cortecchia, C. N. Saggau, S. Martani, G. Folpini, E. Feltri, M. D. Albaqami, L. Ma and A. Petrozza, ACS Nano, 2022, 16, 20671–20679.
2 T. Handa, T. Yamada, M. Nagai and Y. Kanemitsu, Phys. Chem. Chem. Phys., 2020, 22, 26069–26087.
3 A. D. Wright, C. Verdi, R. L. Milot, G. E. Eperon, M. A. Pérez-Osorio, H. J. Snaith, F. Giustino, M. B. Johnston and L. M. Herz, Nat. Commun., 2016, 7, 11755.
Charlie Henderson, Imperial College London
Charge Transfer Complex Formation Between Organic Interlayers Drives Light-Soaking in Large Area Perovskite Solar Cells
Charlie Henderson,a Joel Luke,a Izabela S. Bicalho,b Luiza Correa,b Emily J. Yang,a Martina Rimmele,c Harry Demetriou,d Sandrine Heutz,d Nicola Gasparini,c Martin Heeney,c,e Diego Bagnisb & Ji-Seon Kima
a Department of Physics and Centre for Processable Electronics, Imperial College London, UK
b Oninn, Av. José Cândido da Silveira 2000 – Horto Florestal, Belo Horizonte, Brazil
c Department of Chemistry and Centre for Processable Electronics, Imperial College London, UK
d Department of Materials and Centre for Processable Electronics, Imperial College London, UK
Email: c.henderson21@imperial.ac.uk / ji-seon.kim@imperial.ac.uk
Perovskite solar cells have seen significant advances in power conversion efficiency (PCE) over the last decade. Inverted (p–i–n) structure perovskite solar cells (PSCs) have seen recent rapid increases in their PCEs to over 25% and are approaching those of the best n–i–p devices (~26%).1 This has largely been driven by new self-assembled monolayer hole transport materials, whilst the traditional fullerene/bathocuproine (BCP) bilayer electron transport layers (ETLs) have remained unaltered. Despite its widespread use in both organic and perovskite optoelectronics, the mechanism by which BCP improves the performance of devices is still unclear. In this talk we will discuss our findings on the mechanism by which BCP is involved in efficient electron extraction from fullerene ETLs.2
We have identified a light soaking (LS) effect in large area, blade coated perovskite solar cells, the strength of which is dependent on ETL material used, something we have previously observed in large area organic photovoltaic devices.3 In this work, devices based on evaporated C60 ETLs show fast, weak LS whilst devices incorporating PC61BM show slow, strong LS. We identify the key molecular origins of this LS effect using a combination of surface photovoltage, ambient photoemission spectroscopy, and Raman spectroscopy, integrated with density functional theory simulations. We demonstrate that BCP forms a photoinduced charge-transfer (CT) complex with both C60 and PC61BM. The C60/BCP complex accelerates C60 dimer formation, leading to a favourable cascading energetic landscape for electron extraction and reduced recombination loss. In contrast, the PC61BM/BCP complex suppresses PC61BM dimer formation, meaning that PC61BM dimerisation is not the cause of LS. Instead, it is the slow light-induced formation of the PC61BM/BCP CT complex itself, and, crucially, the new energetic transport levels associated with it, which cause the much slower and stronger LS effect of PC61BM based PSCs. These findings provide key understanding of photoinduced ETL/BCP interactions and their impact on the LS effect in PSCs.
Our work provides important insight into the role of the widely used fullerene/BCP electron transport system in perovskite solar cells and this talk will demonstrate that the optimisation of non-perovskite interfaces in devices is critical for further development of PSCs.
1 S. M. Park, M. Wei, N. Lempesis, W. Yu, T. Hossain, L. Agosta, V. Carnevali, H. R. Atapattu, P. Serles, F. T. Eickemeyer, H. Shin, M. Vafaie, D. Choi, K. Darabi, E. D. Jung, Y. Yang, D. Bin Kim, S. M. Zakeeruddin, B. Chen, A. Amassian, T. Filleter, M. G. Kanatzidis, K. R. Graham, L. Xiao, U. Rothlisberger, M. Grätzel and E. H. Sargent, Nature, 2023, 624, 289–294.
2 C. Henderson, J. Luke, I. S. Bicalho, L. Correa, E. J. Yang, M. Rimmele, H. Demetriou, Y. Chin, T. Lan, S. Heutz, N. Gasparini, M. Heeney, D. Bagnis and J. Kim, Energy Environ. Sci., 2023, 16, 5891–5903.
3 J. Luke, L. Corrêa, J. Rodrigues, J. Martins, M. Daboczi, D. Bagnis and J. Kim, Adv. Energy Mater., 2021, 11, 2003405.
Saiful Islam, University of Oxford
From batteries to solar cells: exploring energy materials on the atomic scale
Further breakthroughs in materials for low carbon energy applications require advances in new compositions and underpinning materials science. Indeed, a greater fundamental understanding into new materials for rechargeable batteries and solar cells require atomic-scale characterization of their structural, electronic and transport behaviour. In this context, combined modelling-experimental work is now a powerful approach for investigating these properties at the atomic scale. This presentation will describe such studies in two principal areas: (i) structural and redox chemistry of lithium-rich cathodes including transition metal layered oxides and disordered rocksalts; and (iii) ion transport and mixed-cation effects in organic-inorganic perovskite compounds for solar cells.
BIO
Saiful Islam has been Professor of Materials Science at the University of Oxford since 2022, before which he was Professor of Materials Chemistry at the University of Bath for 16 years. He grew up in north London and obtained his Chemistry degree and PhD from University College London (under Prof Richard Catlow FRS) followed by a Research Fellowship at the Eastman Kodak Labs, New York, USA.
His research encompasses atomic- and nano-scale processes in new materials for lithium- and sodium-ion batteries, and for perovskite solar cells. He leads the Faraday Institution CATMAT project on lithium cathode materials and has received several awards including the 2022 Royal Society Hughes Medal, the 2020 American Chemical Society Storch Award in Energy Chemistry, and 2017 RSC Peter Day Award for Materials Chemistry.
Saiful presented the 2016 Royal Institution Christmas Lectures for BBC TV on the theme of energy, which included a lemon battery world record. He is a Patron of Humanists UK and when not exploring energy materials, he enjoys family breaks (as a dad of two), films and indie music.
Research website: https://bit.ly/327BgQr and Twitter @SaifulChemistry
Ian Jacobs, University of Cambridge
In-operando spectroscopic characterization of non-equilibrium states in a highly doped polymer
Heavily doped organic semiconductors are critical to a variety of applications, including thermoelectrics, bioelectronics, and neuromorphic computing devices. Recent advances have enabled the routine doping of polymer films to extremely high carrier densities on the order of one charge per polymer repeat unit. In this regime charge transport is comparatively poorly understood due to the non-negligible effects of electron-electron and electron-ion interactions, in addition to the ever-present role of static and transient disorder.
Here, we report on dual-gated indacenodithiophene-co-benzothiadiazole (IDTBT) organic electrochemical transistors (OECTs) and ion-exchange doped films, in which a second field-effect gate enables us to modulate the carrier density independently from the ion density. These devices show unusual behavior; at low temperatures, field effect transfer curves are highly non-linear and in many cases even ambipolar, while at high temperatures a purely linear curve is obtained. Using solid-state NMR and infrared charge modulation spectroscopy, we demonstrate that this non-linearity at low temperatures is a signature of a non-equilibrium state resulting from freeze-out of ionic motion. Under these conditions, we see an enhancement in charge delocalization, electrical conductivity, and Seebeck coefficient, pointing to a new pathway to enhanced thermoelectric performance.
BIO
Ian Jacobs is a Royal Society University Research Fellow in the Optoelectronics Group of the Cavendish Laboratory, University of Cambridge. He holds a B.E. in chemical engineering from The Cooper Union and a Ph.D. in materials science and engineering from the University of California, Davis. His graduate research focused on the use of molecular dopants to control semiconducting polymer solubility and methods for controlling doping in organic semiconductors, with applications to nanoscale patterning. Following his PhD, he was a Royal Society Newton International Fellow in the group of Henning Sirringhaus at the University of Cambridge, where he helped to develop the ion-exchange doping strategy for semiconducting polymers. His current research interests concern the interplay between charge and ion transport in organic electrochemical transistors and doped films, with applications in thermoelectric and electrochromic devices.
Linnea Lind, Imperial College London
Fe-Complexes for Solar Energy Conversion
Nature captures sunlight via light-absorbing molecules. Similarly, photosensitiser molecules can be used in applications of solar cells and artificial photosynthesis to absorb sunlight and create charge-separation. Successful photosensitisers have in the past been based on a Ru polipyridyl scaffold,[1] despite Ru being one of the scarcest elements in Earth’s crust. This work aims to replace Ru by Fe, where the greatest challenge is to prolong the significantly shorter excited state lifetime of Fe-complexes. The excited state lifetime is controlled by the deactivation pathways of the molecule, dictated by the excited state landscape. This work explains how small variations in the ligand structure of an Fe-complex can change the dominating excited state and how to prolong the excited state lifetime from sub-ps to ns by using carbene ligands.[2]
Proof-of-principle demonstrations have shown that Fe carbene complexes can successfully be implemented in solar cells,[3] photocatalysis reactions such as hydrogen production[4] and LEDs.[5] The crucial electron transfer steps in these systems take place on the ps timescale, and are characterised by time-resolved spectroscopy techniques. In particular, this work has shown that the bottleneck for solar cell performance is an ultrafast recombination reaction, that brings a majority of the transferred electrons back to the photosensitiser.[3]
[1] A. Juris & V. Balzani, Ru(II) Polypyridine Complexes: Photophysics, Photochemistry, Electrochemistry and Chemiluminescence, Coordination Chemistry Reviews 84 (1988) 85-277. https://doi.org/10.1016/0010-8545(88)80032-8
[2] L. Lindh et al., Photophysics and Photochemistry of Iron Carbene Complexes for Solar Energy Conversion and Photocatalysis, Catalysts 10 (2020) 315. https://doi.org/10.3390/catal10030315
[3] L. Lindh et al., Dye-Sensitized Solar Cells based on Fe N-heterocyclic Carbene Photosensitizers with Improved Rod-like Push-Pull Functionality, Chemical Science 12 (2021) 16035-16053. https://doi.org/10.1039/d1sc02963k
[4] J. Schwarz et al., High Turnover Photocatalytic Hydrogen Formation with an Fe(III) N-Heterocyclic Carbene Photosensitiser, Chemical Communications 58 (2022) 5351-5354. https://doi.org/10.1039/D2CC01016J
[5] Z. Cui et al., Organic Light-Emitting Diodes Based on an Iron(III) Complex with Doublet Emission, CCS Chemistry 4 (2022) 2953-2958. https://doi.org/10.31635/ccschem.022.202101693
Davide Nodari, Imperial College London
Dark current in broadband perovskite-organic heterojunction photodetectors controlled by interfacial energy band offset
Davide Nodari1,2, and Nicola Gasparini1,2
1Department of Chemistry and Molecular Sciences Research Hub, Imperial College London, White City Campus, 82 Wood Lane, London, W12 0BZ, U.K.
2Centre for Processable Electronics, Imperial College London, South Kensington Campus, London, SW7 2AZ, U.K.
Lead halide perovskite and organic semiconductors have been promising classes of materials for photodetector (PD) applications. However, despite their high performances with low noise and specific detectivity (D*) values exceeding the one of silicon detectors, perovskite PDs working regime is limited to the visible and the first fraction of near-infrared (NIR) spectrum due to their bandgap.1,2 Differently, organic PDs have the advantage of tunable absorption thanks to their chemical design. In this work, we fabricated perovskite-organic heterojunction (POH) PDs with extended absorption up to 900 nm, thanks to the deposition of a donor:acceptor bulk-heterojunction (BHJ) layer on top of the perovskite. We systematically studied the effect of the energetic of the donor materials on the dark current (Jd) of the device by using polymers from the PBDB-T family (PCE12, PM6 and PM7). By using this approach, PM7-based POH delivered ultra-low noise of 2 x 10-14 A Hz-1/2 and high specific detectivity D* of 4.7 x 1012 Jones (-0.5 V) at 840 nm. This was achieved by increasing the energetic barrier between the HOMO of the donor and the LUMO of the acceptor as explained by our analytical model.3 We found that the donor sites effectively act as recombination centres at the perovskite-BHJ interface, affecting Jd. In particular, PM7 with a deeper HOMO level, formed a larger LUMO(Y6)-HOMO(D) gap compared to PM6. This resulted in a smaller contribution of the HOMO of the donor to thermal noise generation, compared to the contribution of the intrinsic traps introduced by Y6 at the perovskite-BHJ interface.
Furlan, Francesco, et al. “Tuning halide composition allows low dark current perovskite photodetectors with high specific detectivity.” Advanced Optical Materials 10.24 (2022): 2201816.
Ollearo, Riccardo, et al. “Vitality surveillance at distance using thin-film tandem-like narrowband near-infrared photodiodes with light-enhanced responsivity.” Science Advances 9.7 (2023): eadf9861.
Sandberg, Oskar J., et al. “Mid-gap trap state-mediated dark current in organic photodiodes.” Nature Photonics 17.4 (2023): 368-374.
Chris Proctor, University of Oxford
Multimodal bioelectronics
Significant advances have been made in the last two decades in interfacing electronic devices with the nervous system. To that end, research efforts are being pursued to develop minimally invasive, implantable bioelectronic devices integrating sensing, stimulating, and dynamic control of geometry. Here we report recent developments towards such multimodal devices for neural interfacing that take full advantage of the favourable properties offered by flexible electronics, conducting polymers and polymer substrates. It is shown that thin, flexible devices can incorporate microfluidic channels to enable new sensing and therapeutic functionalities. One such technology leverages the mixed conducting properties of conducting polymers and molecularly imprinted polymers for real time sensing of cortisol. Furthermore, we show fluidic components can open the door to novel implantation strategies that can reduce the surgical footprint required for implantation of widely used bioelectronic devices. We anticipate this work will accelerate the development of a new generation of bioelectronic devices for diagnostics and therapy.
BIO
Chris Proctor is an Associate Professor in Bioelectronics and BBSRC David Phillips Fellow in the Institute of Biomedical Engineering and Engineering Science Department at the University of Oxford. He leads the Oxford Bioelectronics Laboratory in the Institute for Biomedical Engineering wherein his research is focused on developing bioelectronic systems to improve healthcare and advance bioscience.
Chris received a B.Sc. in Interdisciplinary Physics from the University of Michigan. Following two years as a general scientist at the U.S. Nuclear Regulatory Commission, he earned a Ph.D. in Materials from the University of California, Santa Barbara where he investigated loss mechanisms in organic photovoltaics. Subsequently, Chris was awarded a postdoctoral fellowship from Whitaker International to develop implantable bioelectronic devices for treating neurological disorders in the Bioelectronics Department at the Ecole des Mines de St Etienne. He then joined the University of Cambridge as a Research Associate and Borysiewicz Biomedical Sciences Fellow. In 2020, Chris started as a BBSRC David Phillips Fellow and group leader in the Engineering Department at Cambridge before moving to Oxford in 2022.
Berjaeu Officer, Imperial College London
Rational design of robust mesh bioelectronics for stable physiological interrogation of cardiomyocytes
Berjaeu Officer1,2,3, Dannielle Cox-Pridmore3,4, Fernando Castro3,4, Patrizia Camelliti2, and Yunlong Zhao1,3,*
1 Dyson School of Design Engineering, Imperial College London, London, SW7 2BX, UK
2 School of Bioscience and Medicine, University of Surrey, Guildford, Surrey, GU2 7XH, UK
3 National Physical Laboratory, Teddington, Middlesex TW11 0LW, UK
4 Advanced Technology Institute, University of Surrey, Guildford, Surrey, GU2 7XH, UK
Email: b.officer23@imperial.ac.uk (B.O), yunlong.zhao@imperial.ac.uk (Y.Z.)
Cardiovascular diseases remain a leading cause of morbidity and mortality worldwide, despite significant advancements in treatment, there is an urgent need for novel therapeutic solutions. In vitro engineering of cardiomyocytes (CMs) offers promising avenues for regenerative medicine and drug therapy. However, conventional in vitro methods for culturing CMs often fail to recapitulate the complex phenotype of native cardiac tissue as well as the complex three-dimensional microenvironment of the native myocardium, limiting their translational potential.
To address this challenge, we propose a multidisciplinary approach to enhance the phenotype of CMs cultured in vitro by introducing a mesh bioelectronic scaffold capable of electrically interrogating CMs in both a 2D and 3D culture setting. Initially inspired by neural interface technologies, we developed our own bioelectronic device utilizing photolithography techniques that features a flexible mesh made of gold electrode ribbons coated with a biocompatible polymer (SU-8). Using this device, we established a means of directly interfacing with CMs. This device was successfully able to facilitate the development and direct electrical stimulation of cardiomyocytes. Enhancing the CMs by inducing nuclei elongation both without (+ 27%) and with (+55%) electrical stimulation.
Although the application of a neural bioelectronic device design to CMs acts as a proof of concept, it has become apparent that further optimization would be necessary for cardiac models and revisions of the bioelectronic mesh design would need to be carried out for the specific physiological characteristics of CMs. To enhance the robustness of the mesh bioelectronic device and optimize the mesh scaffold design specifically for CMs, we refined the selected ribbon widths (30-60µm), reducing the spacing between ribbons for better cell proximity, and increasing device thickness for enhanced stiffness and handling. These modifications have significantly improved cell-to-device interaction, promoting cell elongation and attachment. The device’s electrical stimulation capabilities have been shown to triple the CMs’ beating rate, closely resembling the contractility of native cardiac tissue. The improved CM functionality resulting from the mesh design and electrical stimulation was thoroughly evaluated using techniques such as immunostaining and optically mapping calcium transients. This optimized bioelectronic platform shows promise in creating accurate cardiac tissue models for regenerative medicine, potentially offering a new avenue for cardiovascular disease therapy.
Martina Rimmele, Imperial College London
Soluble Conjugated Polymers without Side Chains: Macrocycles as Comonomers
Martina Rimmele,a Troy L. R. Bennett,a Martin Heeney,a,b Florian Glöcklhofera ,ca
a Department of Chemistry and Centre for Processable Electronics, Imperial College London, London, W12 0BZ, United Kingdom
b King Abdullah University of Science and Technology (KAUST), KAUST Solar Centre (KSC), Physical Sciences and Engineering Division (PSE), Thuwal, 23955 6900 Saudi Arabia
c Institute of Applied Synthetic Chemistry, TU Wien, Getreidemarkt 9/163, 1060 Vienna, Austria
Solubility is pivotal in conjugated polymer synthesis and application, facilitating thin film fabrication via solution based techniques. 1 While aliphatic side chains enhance solubility, they can compromise charge carrier mobility, crystallinity, and optical properties, necessitating meticulous optimization. 2 Macrocycle incorporation of fers a promising alternative, potentially enhancing solubility without sacrificing performance. Reports suggest macrocycles can improve solubility and act as redox active units, like in PCT and its derivatives, offering excellent redox properties. 3 Hence, w e aimed to integrate macrocycles into conjugated polymer backbones to sidestep the drawbacks of aliphatic side
chains. Utilizing PCT units, we copolymerised them with (bi)thiophene derivatives.
In this work we present a new class of materials, conjugated polymers with macrocycles incorporates a s comonomers. The polymers are soluble in conventional solvents, and we characterised the ir optoelectronic properties. We furthermore studied the molecular structure s using scanning tunnelling microscopy (S TM) to get information about the molecular weight and structural confirmation.
Due to their unique structure and properties, we believe that these are a n interesting class of materials and th at the incorporation of conjugated macrocycles into conjugated polymers is a useful strategy to prepare soluble materials.
- Forrest, S. R., The path to ubiquitous and low cost organic electronic appliances on plastic. Nature 2004, 428 (6986), 911 918.
- Yang, Y.; Liu, Z.; Zhang, G.; Zhang, X.; Zhang, D., The Effects of Side Chains on the Charge Mobilities and Functionalities of Semiconducting Conjugated Polymers beyond Solubilities. Advanced Materials 2019, 31 (46),
- Eder, S.; Yoo, D. J.; Nogala, W.; Pletzer, M.; Santana Bonilla, A.; White, A. J. P.; Jelfs, K. E.; Heeney, M.; Choi, J. W.; Glöcklhofer, F., Switching between Local and Global Aromaticity in a Conjugated Macrocycle for High Performance Organic Sodium Ion Battery Anodes. Angewandte Chemie International Edition 2020, 59 (31), 12958 12964.
David O Scanlon, University of Birmingham
Computational prediction and experimental realisation of new transparent conducting oxides
Transparent conducting oxides have become ubiquitous in modern optoelectronics. However, the number of oxides that are transparent to visible light and have the metallic-like conductivity necessary for applications is limited to a handful of systems that have been known for the past 40 years. In this work, we use hybrid density functional theory and defect chemistry analysis to demonstrate that tri-rutile zinc antimonate, ZnSb2O6, is an ideal transparent conducting oxide and to identify gallium as the optimal dopant to yield high conductivity and transparency. To validate our computational predictions, we have synthesized both powder samples and single crystals of Ga-doped ZnSb2O6 which conclusively show behaviour consistent with a degenerate transparent conducting oxide. Our work demonstrates the possibility of a family of Sb(V)-containing oxides for transparent conducting oxide and power electronics applications.
BIO
Professor David O. Scanlon completed both his undergraduate training in Computational Chemistry (2002-2006) and his PhD in Chemistry (2006-2011) at Trinity College Dublin, with his postgraduate research conducted under the tutelage of Prof Graeme W. Watson. He was awarded the Royal Irish Academy Young Chemists prize for 2011, for “the most outstanding Irish PhD thesis of 2011 in the area of the chemical sciences”. In 2011 he obtained a prestigious Thomas Young Centre Junior Research Fellowship, which funded a collaborative visit to UCL to work with Professor Sir Richard Catlow, FRS. He then held the position of Ramsay Memorial Fellow in the Department of Chemistry in University College London (UCL) from 2011 to 2013. In September 2013 he was appointed to a lectureship in Computational, Inorganic and Materials Chemistry, jointly held between UCL and Diamond Light Source, the UK’s national synchrotron. He was promoted to Reader (Associate Professor) in 2016, and to Full Professor of Computational Materials Design in 2018, only 7 years after the award of his PhD. In 2023, he took up the Chair in Computational Chemistry at the University of Birmingham, and will lead a multi-million pound investment into High Performance Computing at the university.
He has published over 290 publications in the top journals in my field, including Nature Materials, Nature Photonics, Energy & Environmental Science, Journal of the American Chemical Society, Physical Review Letters, Chemical Science, Advanced Materials etc. His work has been cited over 23,000 times and has earned an H-index of 85 (Google Scholar). In 2015 he was awarded the Harrison Meldola Memorial Prize from the Royal Society of Chemistry (RSC) for the “development and application of computational techniques to understanding and predicting the properties of functional semi-conductors for energy applications”, and was made a Fellow of the RSC. In 2021, he was awarded the inaugural RSC Materials Chemistry Division Early Career Award for “contributions to materials modelling of conducting oxides”. He has raised over £17 Million in research funding since 2016 from funders such as the Engineering and Physical Sciences Research Council (UKRI – EPSRC), the ERC, the Faraday Institution and Innovate UK. He was named the inaugural Lead Editor of PRX Energy, a new fully open access and highly selective multidisciplinary energy journal from the American Physical Society, and now sits on the Council of Lead Editors for the APS. He also sits on the Editorial Advisory Boards for Materials Horizons, Journal of Materials Chemistry C, Materials Advances, Chemical Communications, ACS Applied Energy Materials, Matter and Cell Reports Physical Science. He also believes in supporting our community; he chairs the Faraday Institution High Performance Computing Committee, sits on the Royal Society International Exchanges Panel and Research Project Panel, and is a member of the RSC Energy Sector Committee. From 2021-2023 he sat on the RSC Materials Chemistry Division Prize Committee, and from 2016-2020 he was the honorary treasurer of the RSC Solid State Chemistry Group. In 2023, he Co-Chaired the flagship RSC Materials Chemistry Division Conference, Materials Chemistry 16 (MC16) in Dublin Ireland.
Seung-Jae Shin, Imperial College London
Post.doc, Department of Material Science and Engineering, Yonsei University
Department of Materials, Imperial College London
E-mail: ssj2515@yonsei.ac.kr
Electrochemistry deals with the physicochemical phenomena at the electrified electrode–electrolyte interface. Although there exist many experimental measurements techniques, it is challenging to investigate the electrochemical interface in atomic resolution.
Computational chemistry has improved the understanding level throughout the all divisions of chemistry. I have developed the QM/MM method to investigate the electrochemical interface and applied the method to study various electrochemical interfaces1–5. The first study is the most fundamental concept in the electrochemistry that is understanding the aqueous electric double layer (EDL) structure. Although it is oldest concept but its structural understanding is still vague that the QM/MM simulation unveils the atomic details of it. The EDL has an important role in many applications including electrochemical catalytic reactions or energy storage systems1. In the following studies, the QM/MM simulation is employed to understand the mechanism of electrochemical CO2 reduction reaction2, electrolytes effect to improve the stability of Li-ion battery cathode4 or Zn-ion battery anode3. Recently, it is also used to investigate the microscopic origin of EDL capacitance with two-dimensional metal organic framework (MOF) electrodes5.
In this seminar, I’ll briefly introduce the methodology developments, and summarise the research using this method.
Shin, S.-J.; Kim, D. H.; Bae, G.; Ringe, S.; Choi, H.; Lim, H.-K.; Choi, C. H.; Kim, H. On the Importance of the Electric Double Layer Structure in Aqueous Electrocatalysis. Nat. Commun. 2022, 13 (1), 174. https://doi.org/10.1038/s41467-021-27909-x.
(2) Shin, S.-J.; Choi, H.; Ringe, S.; Won, D. H.; Oh, H.-S.; Kim, D. H.; Lee, T.; Nam, D.-H.; Kim, H.; Choi, C. H. A Unifying Mechanism for Cation Effect Modulating C1 and C2 Productions from CO2 Electroreduction. Nat. Commun. 2022, 13 (1), 5482. https://doi.org/10.1038/s41467-022-33199-8.
(3) Kim, M.; Shin, S.; Lee, J.; Park, Y.; Kim, Y.; Kim, H.; Choi, J. W. Cationic Additive with a Rigid Solvation Shell for High‐performance Zinc Ion Batteries. Angew. Chem. Int. Ed. 2022, 61 (47), e2022115. https://doi.org/10.1002/anie.202211589.
(4) Oh, H.; Shin, S.-J.; Choi, E.; Yamagishi, H.; Ohta, T.; Yabuuchi, N.; Jung, H.-G.; Kim, H.; Byon, H. R. Anion-Induced Interfacial Liquid Layers on LiCoO 2 in Salt-in-Water Lithium-Ion Batteries. JACS Au 2023, jacsau.3c00061. https://doi.org/10.1021/jacsau.3c00061.
(5) Shin, S.-J.; Gittins, J. W.; Golomb, M. J.; Forse, A. C.; Walsh, A. Microscopic Origin of Electrochemical Capacitance in Metal-Organic Frameworks. ChemRxiv 2023, preprint. https://doi.org/10.26434/chemrxiv-2023-p0wvm.
Nuria Tapia-Ruiz, Imperial College London
Building and understanding the behaviour of high-performance batteries by rationalising structural electrode design
In this talk, I will discuss several examples where through materials’ rational design across relevant length scales we have produced electrode materials with desirable electrochemical properties for different battery technologies, such as cycling stability and high energy and power densities. Furthermore, I will cover the importance of a multi-technique approach to understanding structure-property relationships in these materials. Finally, I will outline future research directions and challenges ahead for the implementation of these materials in battery technology.
BIO
Nuria graduated in Chemistry from the University of Barcelona. After this, she pursued her PhD studies in hydrogen storage materials with Prof. Gregory at the University of Glasgow. She then moved to the University of St. Andrews to work as a Postdoctoral Fellow with Prof. Bruce in energy storage materials. In 2014, she moved with Prof. Bruce and his research group to Oxford (Materials Department, University of Oxford) to work as a Research Fellow where she took up the role of Team Leader of the Sodium-ion battery team. She started her independent career as a Lecturer in Chemistry at Lancaster University in 2017 and was promoted to Senior Lecturer in 2020. In November 2022, her research group and lab moved to Imperial College London where she is Senior Lecturer. Dr Tapia-Ruiz has been the recipient of some awards including, the SRUK Emerging Talent Award (2022), the Lancaster Researcher of the Year Award (2021), the Royal Society of Chemistry mobility Award (2019), the CAMS Fellowship Award (2019), the STFC Batteries Proof of Concept Award (2017), the STFC Batteries Experimental Design Award (2016), the Elizabeth Aitken Award (2011), and the Rotary Club Award (2003).
Amita Ummadisingu, University College London
Multi-length scale structure of two-dimensional halide perovskites
Organic-inorganic perovskites are hybrid materials which have been found to possess excellent optoelectronic properties, making them highly suitable for applications such as solar cells, light-emitting diodes, and thin film field-effect transistors [1]. In recent years, two-dimensional (2D) perovskite structures offering higher tunability of physical properties and new capabilities are emerging as promising candidates for incorporation into these devices [2]. However, structural aspects of these 2D perovskites in phases such as the Dion–Jacobson (DJ) are not yet well understood.Here, we present a systematic approach to the characterization of new 2D perovskites, taking DJ structures based on 1,4-phenylenedimethanammonium (PDMA) as an example. The single crystal structure of a DJ phase based on PDMA is presented and high-field solid-state NMR spectroscopy is used to characterize the structure of PDMA-based iodoplumbates prepared as thin films and bulk microcrystalline powders. We find that their atomic-level structure does not depend on the method of synthesis and that it is ordered and similar for all iodoplumbate homologues. Moreover, the presence of lower (n) homologues in thin films is identified through UV–Vis spectroscopy, photoluminescence spectroscopy, and X-ray diffraction measurements. A closer look using cathodoluminescence mapping shows that the micron-scale microstructure corresponds to a mixture of different layered homologues that are well distributed throughout the film and the presence of layer edge states which dominate the emission. This work reveals the formation of DJ phases based on PDMA as the spacer cation and their properties on a multi-length scale, which is relevant for their practical application in various optoelectronic devices [3].
1.M. A. Green, A. Ho-Baillie, H. J. Snaith, The Emergence of Perovskite Solar Cells. Nature Photonics 2014, 8, 506-514.
2.L. Mao et al., Hybrid Dion−Jacobson 2D Lead Iodide Perovskites. JACS 2018, 140, 3775-3783.
3.A. Ummadisingu et al., Multi-Length Scale Structure of 2D/3D Dion–Jacobson Hybrid Perovskites Based on an Aromatic Diammonium Spacer. Small 2022, 18, 2104287.
BIO
Amita Ummadisingu holds a Dr.Sc. in Chemistry and Chemical Engineering from the Ecole polytechnique fédérale de Lausanne (EPFL), Switzerland, where she worked with Prof. Michael Grätzel on unravelling the formation of hybrid perovskite materials for highly efficient solar cells. In 2019, she joined the University of Cambridge, UK as a Swiss National Science Foundation Fellow, hosted by Prof. Henning Sirringhaus. She was looking into the charge transport physics of perovskite thin films, particularly ion migration. In 2022, she joined the Department of Chemical Engineering at UCL as a Lecturer in Nature-inspired Chemical Engineering. Her current research is focused on understanding and harnessing natural phenomena on the nanoscale for the engineering and design of efficient optoelectronic devices which are compatible with scale-up for manufacturing.
Ming Xu, Imperial College London
Minimally Invasive Power Supply with Long-term Stability for Implantable Electronics
Ming Xu1,2, and Yunlong Zhao1,3,*
1 Dyson School of Design Engineering, Imperial College London, London, SW7 2BX, UK
2 Advanced Technology Institute, University of Surrey, Guildford, Surrey, GU2 7XH, UK
3 National Physical Laboratory, Teddington, Middlesex TW11 0LW, UK
Email: m.xu24@imperial.ac.uk (M.X), yunlong.zhao@imperial.ac.uk (Y.Z.)
As various bioelectronics systems developed for monitoring biological signals and therapy have been extensively explored and deployed clinically, surging demand for minimally invasive electronics with good adaptation to 3D dynamically curved bio interfaces occurred in recent years. To realise the conformable application of these advanced electronics at curved bio-interfaces and sustained operation of bioelectronics systems, a miniaturised power supply system with enhanced performance and good long-term stability is urgently needed. Rechargeable flexible energy storage devices integrated with wireless energy harvesting technology can provide reasonable solutions to enable sustainable stable bioelectronics systems.[1] Herein, we present two strategies for minimally invasive power supply. Firstly, we propose an injectable flexible needle-like micro supercapacitor with high energy density for micro LED (optogenetic therapy) and sensors.[2] The fabricated micro supercapacitor utilised activated carbon mixed with multiwall carbon nanotubes as electrode materials to increase the capacitance and employed water-in-salt electrolyte to increase the working voltage from 1V to 1.8V, it then can provide high and stable power output and be integrated with functional electronics such as micro-LEDs and pressure sensors, which could be injected into the targeted tissue areas to realise biomedical purposes with miniaturised incision. Secondly, to further minimise the invasion and avoid the pain caused by the implantation procedure of bioelectronics integrated with minimally invasive power system, we proposed a programmable Bio-LED integrated with sustainable and flexible wireless rechargeable micro supercapacitor for optogenetic therapy. Benefiting from photolithography microfabrication, the micro-coil and micro supercapacitor were integrated into a flexible thin film substrate with the micro LED circuit. It can harvest wireless power to charge the supercapacitor with high specific capacitance and good stability after 10000 cycles. As an application, the system can provide a voltage of up to 4V and power the micro LED to continuously work wirelessly under the epidermal area.[3] Furthermore, after being connected with integrated circuit chips, the Bio-LED could achieve programmable pulse light for specific optogenetic purposes powered by the flexible wireless rechargeable system, which was proved to be biocompatible in cell culture and functional stably when immersed in PBS solution for a long period. In conclusion, we provide promising strategies for miniaturized minimally invasive power supply systems with enhanced performance, good long-term stability and monolithic all-in-one design for implantable biomedical electronics. In the longer term, it is expected to provide new possibilities and bring about substantial advancements in sustained disease-diagnosis capabilities and biological therapies.
[1] Xu M, Yang K*, Zhao Y* et al. Minimally invasive power sources for implantable electronics[C]//Exploration. 2024, 4(1): 20220106.
[2] Injectable flexible needle-like micro supercapacitor with high energy density (In preparation).
[3] Programmable Bio-LED integrated with sustainable wireless rechargeable micro supercapacitor for optogenetics. (In preparation)
Gong Yi, Imperial College London
Ampere-hour-scale soft-package potassium ion hybrid capacitors enabling 6-minute fast-charging
Yi Gong 1,2, et al. and Yunlong Zhao 1,3*
1 Dyson School of Design Engineering, Imperial College London, London, SW7 2BX, UK
2 Advanced Technology Institute, University of Surrey, Guildford, Surrey, GU2 7XH, UK
3 National Physical Laboratory, Teddington, Middlesex TW11 0LW, UK
Email: y.gong24@imperial.ac.uk(Y. G.), yunlong.zhao@imperial.ac.uk (Y.Z.)
Extreme fast charging of Ampere-hour (Ah)-scale electrochemical energy storage devices targeting charging times of less than 10 minutes are desired to increase widespread adoption. However, this metric is difficult to achieve in conventional Li-ion batteries due to their inherent reaction mechanism and safety hazards at high current densities. In this work, we report 1 Ah soft-package potassium-ion hybrid supercapacitors (PIHCs), which combine the merits of high-energy density of battery-type negative electrodes and high-power density of capacitor-type positive electrodes. The PIHC consists of a defect-rich, high specific surface area N-doped carbon nanotube-based positive electrode, MnO quantum dots inlaid spacing-expanded carbon nanotube-based negative electrode, carbonate-based non-aqueous electrolyte, and a binder- and current collector-free cell design. Through the optimization of the cell configuration, electrodes, and electrolyte, the full cells (1 Ah) exhibit a cell voltage up to 4.8 V, a high full-cell level specific energy of 140Wh kg−1 (based on the whole mass of the device) with a full charge of 6 minutes. An 88% capacity retention after 200 cycles at 10C(10A) and voltage retention of 99% at 25 ± 1 °C are also demonstrated.