In 2019, the Faculty of Engineering awarded 10 PhD studentships as part of its transition to a sustainable zero-pollution economy strategy. Funding for the studentships comes from the EPSRC DTP, industry partners and departments. The students are all aligned with the NERC-funded Science and Solutions for a Changing Planet DTP run by the Grantham Institute. Find out more about the students and their projects below.
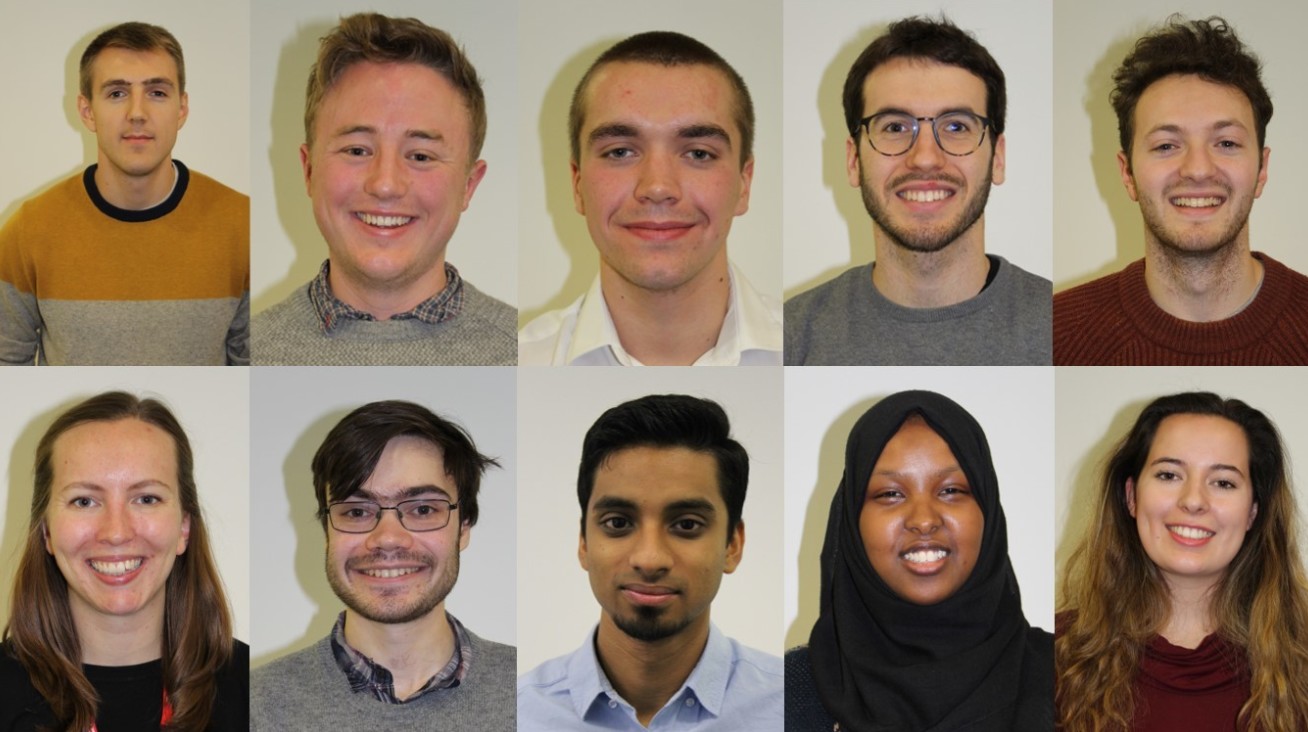
Project summaries
- Marcus Annegarn - Understanding the atomic and electronic structure of particulate matter from theory and experiment
- Alex Bowles - Development of activated carbons from End-of-life Tyre rubber Char for Carbon Capture and storage, for application in the global cement
- Ethan Errington - Engineered Lyophilic Nanoparticles for Fat Recovery from Waste Streams
- Albert Fabregas-Flavia - Building tools and technologies for self-regulated nitrogen fixation in plant-associated bacteria
- Daniel Greenblatt - Transition to zero-pollution transport through optimal hybrid powertrains
- Catrin Harris - Advanced petrophysics for the characterisation of trapping mechanisms for CO2 storage in the subsurface
- Enrico Manfredi Haylock - A fundamental study of deep eutectic solvents and their application to green battery recycling processes
- Waseem Marzook - Thermal performance and low temperature degradation of Li-ion batteries
- Amran Mohamed - Polymeric Additives in Lubricants for Electric Vehicle Powertrains
- Charlotte Roe - BatBath - Immersion cooling of lithium-ion batteries with dielectric fluids
Supervisors: Dr Johannes Lischner and Professor Alexandra Porter, Department of Materials
The goal of this PhD project is to gain insights into the atomic and electronic structure of particulate matter (PM) using a combination of accurate first-principles calculations and state-of-the-art transmission electron microscopy measurements and to correlate these insights with adverse health effects in the lung. The resulting detailed mechanistic understanding will pave the way towards a targeted approach for mitigating the effects of pollution, for example via improved catalysts or green infrastructure.
Supervisor: Dr Geoff Fowler, Department of Civil and Environmental Engineering
Industrial Partner: Pyrenergy Ltd
This PhD research project proposes to develop novel, modified activated carbon materials, using feedstock extracted from waste tyre rubber. The properties of this material would be optimised to be applied for the adsorption of carbon dioxide emitted from the manufacture of Ordinary Portland Cement (OPC). Through using tyres as a feedstock to manufacture activated carbon with bespoke surface and porosity properties, this research will reduce the impact associated with the pollution and health effects from waste tyres. Additionally, this project will develop a bespoke material specifically designed to address a major source of carbon dioxide. The consequences of the uncontrolled release of CO2 will have significant implications for the long term stability of the climate and ultimately the survival of global ecosystems.
Supervisors: Professor Jerry Heng and Dr Miao Guo, Department of Chemical Engineering
Industrial Partner: Scottish Water
Rapid urbanisation in major cities due to economic growth brings about a number of major challenges in particular ongoing environmental degradation. A unique opportunity exists to develop an innovative technology and implement strategies in the treatment of waste for the recovery of valuable products. In this PhD research project, we propose to develop a technology for the recovery of value added products (resource circular economy) from domestic waste (zero pollution). Specifically, this PhD programme will develop novel Engineered Lyophilic Nanoparticles (ELN) by incorporating lyophilic moieties for the adsorption of fats, for its removal and recovery, from domestic wastewater. The performance and adsorption capacity of ELNs will be experimentally determined, to obtain both thermodynamic and kinetic data for single and multiple cycles. These experimental data will serve as input to model based process design and optimisation to enable economic feasibility and technology performance evaluation.
Supervisor: Professor Guy-Bart Stan, Department of Bioengineering
Nitrogen fertilisers are at the heart of modern agriculture and have contributed decisively to feed the rapidly growing global population over the past 50 years. With this rapidly growing population expected to reach 9.8 billion by 2050, N fertilisers are likely to play an equally key role in ensuring global food security in the next decades. And yet, their use poses a serious threat to human health and the environment, mainly in the form of nitrous oxide emissions, groundwater contamination and eutrophication of freshwater ecosystems. In this context, microbial inoculants have emerged as an exciting alternative to reduce N pollution from agricultural systems. Microbial inoculants have indeed shown great promise in laboratory and greenhouse experiments, but results in the field have so far been inconsistent, in part because of the inability of the inoculants to compete with the indigenous microbial communities that inhabit the rhizosphere. In this project, we will use a combined synthetic biology and control engineering approach to bridge the existing gap between the performance of these microbial inoculants under laboratory and real field conditions, with the ultimate goal of developing more robust microbial inoculants for sustainable agriculture.
Supervisor: Professor Peter Lindstedt, Department of Mechanical Engineering
Industrial Partner: Toyota Motor Europe
The transition to a zero-pollution society necessitates novel improvements in vehicle powertrain design, in order to both reduce emissions and increase thermal efficiency. Additionally, the non-renewable source of vehicle fuels has caused interest in the increased use of alternative bio-derived fuels. Historically, apart from in advanced defence and motorsport applications, the properties of a fuel have been treated independently of the energy conversion device. This project, in collaboration with Toyota, a leading hybrid powertrain designer, seeks to remove this limitation through the determination and study of optimal fuel components. Conventional fuel performance descriptors are inadequate for assessing suitability in advanced hybrid powertrains. Through a dual approach of direct experimental measurement of critical parameters and the translation of this data into computational models, various fuel components of interest will be assessed. In doing so, the correlation of fuel structure with performance descriptors, these descriptors and powertrain performance and the potential for the application of machine learning techniques will be studied.
Supervisors: Professor Ann Muggeridge and Dr Sam Krevor, Department of Earth Science and Engineering, and Alistair Jones, BP & Imperial College London
Industrial Partner: BP
Carbon Capture and Storage (CCS) is the process by which CO2 is captured and permanently stored deep within the subsurface. Incorporating CCS technology with fossil fuel energy production, and other emissions sources difficult to eliminate, reduces overall mitigation costs and increases flexibility in achieving a net zero-carbon society. In combination with direct air capture CCS is a negative emissions technology. Geological carbon storage uses physical and chemical trapping mechanisms to permanently sequester CO2 in the subsurface. In the long-term, secondary trapping mechanisms become increasingly important to storage security. The focus of this project is understanding capillary and dissolution trapping, key trapping mechanisms immobilising a significant proportion of the CO2 plume. The method uses experimental core floods with X-ray imaging at two scales (micro CT and medical CT), combined with numerical modelling. The aim is to evaluate the impacts of rock heterogeneity on upscaled capillary trapping and to develop a constitutive law to represent the rate of interphase mass transfer. The ability to model and predict trapping over large spatial scales in complex geological systems is essential for storage security.
Supervisor: Dr David Payne, Department of Materials
Industrial Partner: EnviroWales Ltd
The aim of this project is to develop a safer and cheaper alternative to the current pyrometallurgical processing method applied to the recycling of lead acid batteries. This will be done by developing a solvent based, low temperature process. The process will use low cost, sustainable and biodegradable solvents to dissolve a variety of lead compounds found in waste battery streams. Dissolved lead can then be recovered chemically or electrochemically as lead oxide or metal, both of which are useful materials for the manufacture of new batteries. This technology could be well positioned to replace the improvised furnaces used by informal battery recyclers that pose a significant pollution hazard in developing countries.
Supervisor: Dr Monica Marinescu, Department of Mechanical Engineering
Industrial Partner: Williams Advanced Engineering
Fast charging of lithium ion batteries is one of the most sought-after features. Key to achieving fast charging is an efficient thermal management of battery cells, in order to prevent overheating and minimise long term degradation that reduces the life of the battery. This PhD focuses on developing a standard thermal metric, the Cell Cooling Coefficient, for cylindrical cells. This form factor is popular with the automotive industry, but for it the relationship between thermal and electrical effects is complex. Apart from heat-induced degradation, Lithium plating is the most important degradation mode associated with fast charging. Lithium plating is notoriously difficult to accurately detect in-operando and to quantify.
Better detection methods for this degradation mode will be developed as part of this project, as well as better optimised thermal management approaches, aimed to reduce plating, delivering safer and longer lasting batteries. This will be achieved by experimentally studying the long term performance of batteries under different thermal management systems, focussing on investigating signs of lithium plating via the cell cooling coefficient metric.
Supervisor: Dr Janet Wong, Department of Mechanical Engineering
Industrial Partner: Shell
The use of only one fluid for the whole powertrain means the fluid would need to achieve right viscosities at various parts of the powertrains: low viscosity at battery pack and motor, which can reach up to 180°C, for effective cooling; and higher viscosity at the drivetrain (room temperature). Note lubricating gears in drivetrains means the rheology of the fluid is mainly regulated by using relatively low molecular weight viscosity modifiers (VMs), which makes achieving rheology/viscosity control in such a wide temperature range in EV lubricant challenging. In this project, we target low molecular weight polymeric viscosity modifiers (VMs) that regulate the rheology of EV lubricants over a wide range of temperatures and shear rates. Being polymeric, their wide varieties of chemistry and molecular architectures provide flexibility to obtain properties tailored to specific applications. This project will use computer simulations to investigate the effect of VM molecular architectures on their stability and behaviour in lubricants in ranges of temperature, shear rates, and contact surfaces encountered in EV. Dissipative particle dynamics (DPD) simulations will be used. DPD method is a coarse grain method that allows simulations to be conducted in length scale and time scale that are close to those encountered in tribological contacts. The goal is to establish relationship among molecular architecture of additives, their stability and effectiveness. The knowledge can that be used to guide VM design for EV lubricants. Focusing on the effect of architecture at different shear rates and temperatures, the project involving investigating: 1. the stability, potential aggregation and confirmation of additives in bulk conditions without shear; 2. surface adsorption of additives on various walls; 3. the effect of shear rate on the stability and conformation of the VMs in bulk solutions and near the wall. Keeping the chemistry constant, the effect of molecular weight, polydispersity, VM-wall interactions and copolymer structures, mixtures of structures on their effectiveness will be examined.
Supervisor: Dr Billy Wu, Dyson School of Design Engineering
Industrial Partner: Shell
As the UK transitions to hybrid and electric vehicles by 2035 it is more important than ever to ensure that the lithium ion (li-ion) batteries used in these cars are working at their optimal performance for the longest time possible. Modern electric vehicles currently use direct cooling (air cooling) and indirect cooling (liquid cooling) methods to cool cells down. Both these methods have their advantages and drawbacks, one of the drawbacks is non uniform temperature distribution within cells and battery packs. This leads to variation in the performance and therefore the lifetime of cells in battery packs.
This project aims to investigate immersion cooling, a novel method for cooling batteries which involves immersing the battery in a dielectric fluid. Immersion cooling has the potential to simplify the cooling system for electric vehicle batteries. The primary purpose for this project is to investigate the effects of immersion cooling on the lifetime and performance of li-ion batteries through both experimental and numerical methods. Cylindrical 18650 cells in immersion fluid will be modelled using COMSOL Multiphysics to investigate the internal thermal gradients and modes of degradation which occur in the cell during discharge and lead to a compromised performance and lifetime. This model will be experimentally validated with different single-phase immersion fluids.