Theme overview
Multiphase Transport Processes addresses problems across varying scales by understanding the behaviour of fluids in application areas which include energy, manufacturing, healthcare and the environment.
Researchers focusing on multiphase transport flows will tackle some of society’s greatest issues in areas such as energy, manufacturing and healthcare.
Examples include the breakup of fluid jets, the motion of liquid droplets over solid walls and liquid films in chemical reactors, condensers and separators, the trapping of supercritical carbon dioxide in subsurface rocks, to flow regime transitions in pipeline transportation, in-body flows within tissue, the circulatory system and the GI tract, complex fluid behaviour in manufacturing plants, and radiative thermal hydraulics in nuclear reactors.
The extremely complex nature of multiphase flows means they are often hard to predict and to probe experimentally which can be problematic as small changes to flows on a molecular level could create large-scale impacts.
The Multiphase Transport Flows research theme aims to address these issues by understanding more about how fluids of varying properties behave.
Through multidisciplinary research, using theoretical, experimental, and numerical simulations, researchers use their understanding of these systems to propose solutions to problems of central importance in industrial, environmental, and biomedical applications.
Related centres and institutes
Objectives and twitter feed
Highlight videos
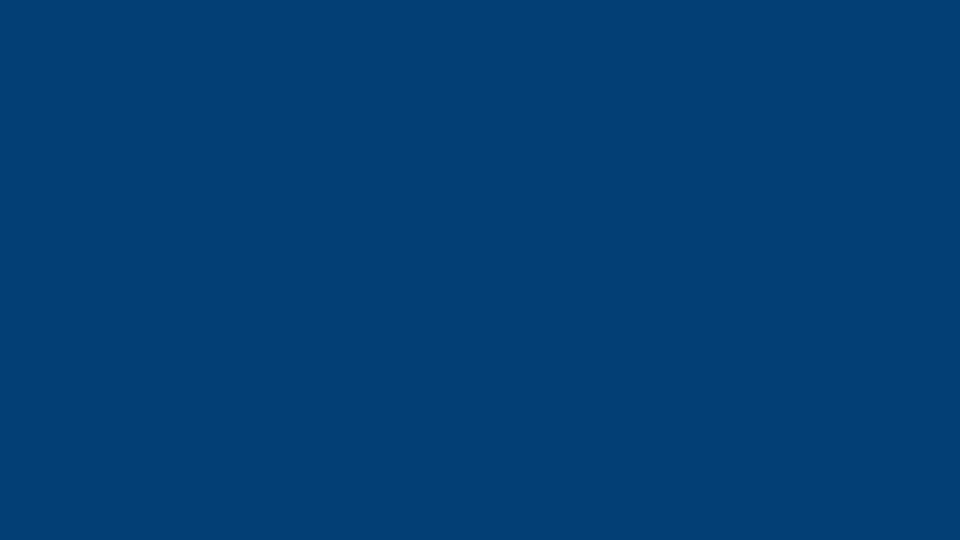
Immersive VR teaching for Fluid Dynamics at Imperial College
Researchers at Imperial have developed virtual reality software for teaching fluid dynamics.
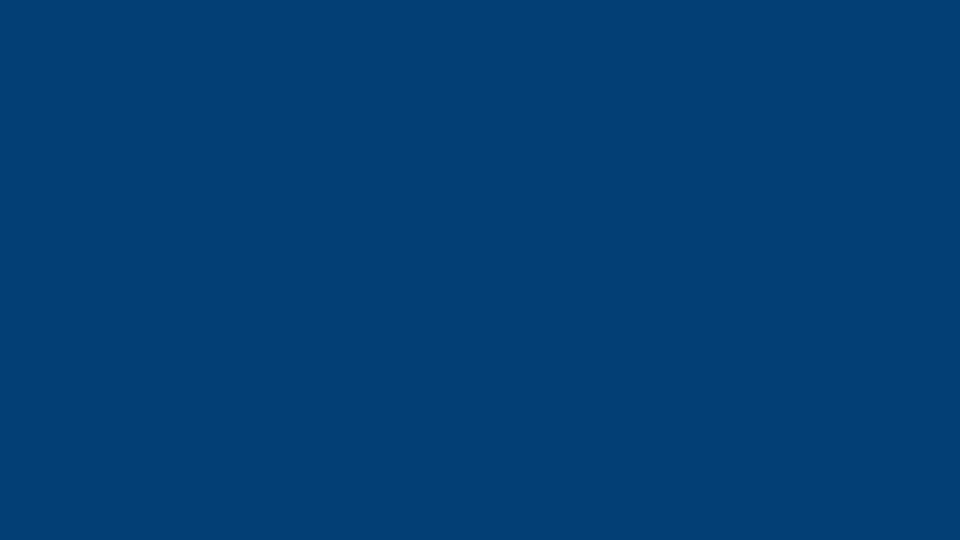
Molecular Fluid Dynamics
Molecules bouncing, jiggling, and flowing together.
Everything is made of molecules; and fluids are no exception. The motion of a fluid can be understood in terms of the collective motion of its molecules bouncing, jiggling, and flowing together. In this video, we take a look at the molecular origins of many well-known phenomena, including the flow of a fluid at the nano-scale, the collective motions which lead to turbulent-like structures, the formation of droplets, the impact of introducing chemicals like soap (a surfactant) and the molecular origins of bubbles in boiling phenomena.
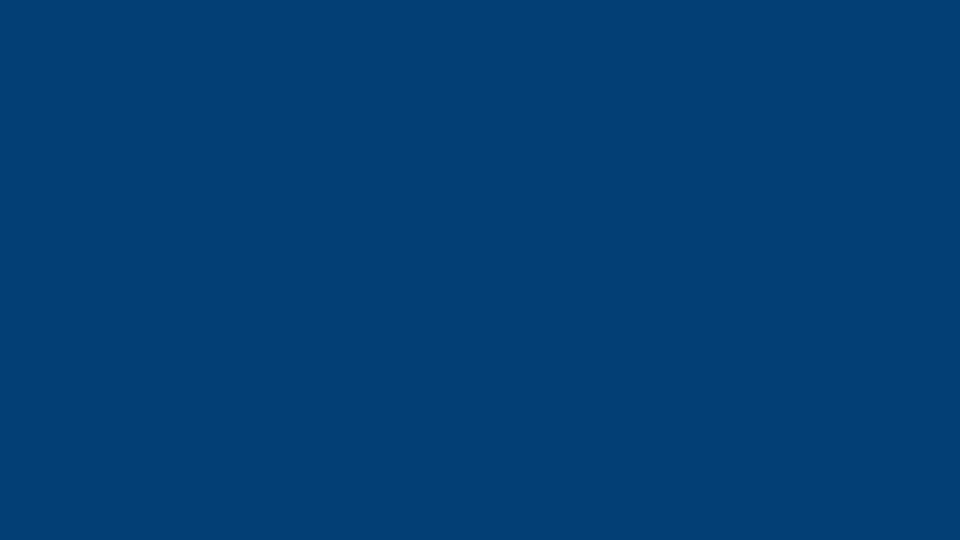
3D DNS of spray formation with the Code BLUE
3D DNS of spray formation with the Code BLUE
Three dimensional direct numerical simulation liquid jet surrounded by a faster coaxial air flow using 2048 cores in an IBM BlueGene/Q machine.
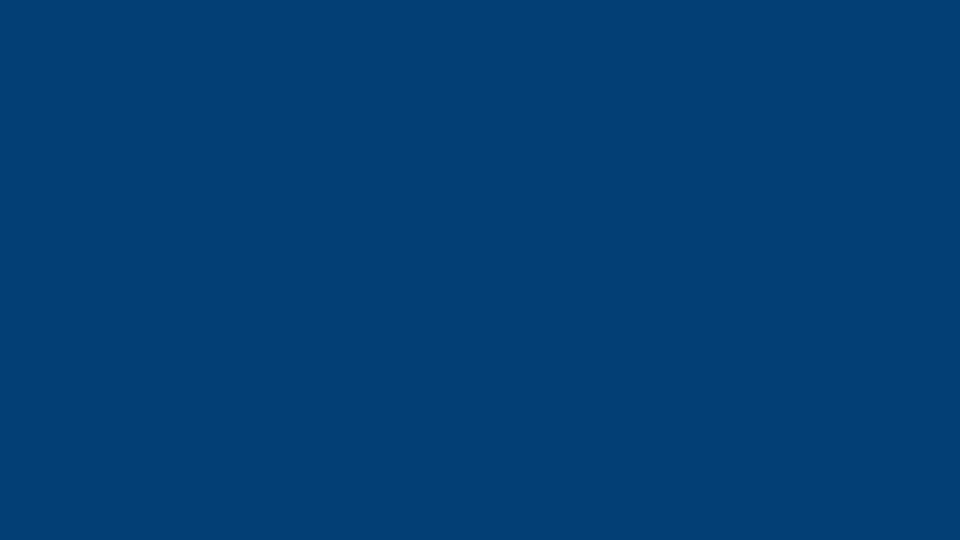
Shaking drop DNS with BLUE
A vibrated drop constitutes a very rich physical system, blending both interfacial and volume phenom
A vibrated drop constitutes a very rich physical system, blending both interfacial and volume phenomena. A remarkable experimental study was performed by M. Costalonga (PhD. Université Paris Diderot, 2015) highlighting sessile drop motion subject to horizontal, vertical and oblique vibration. Several intriguing phenomena are observed such as drop walking and rapid droplet ejection. We perform three-dimensional direct numerical simulations of vibrating sessile drops where the phenomena described above are computed using the massively parallel multiphase code BLUE. The fluid interface solver is based on a parallel implementation of a hybrid Front Tracking/Level Set method designed to handle highly deforming interfaces with complex topology changes. We developed parallel GMRES and multigrid iterative solvers suited to the linear systems arising from the implicit solution for the fluid velocities and pressure in the presence of strong density and viscosity discontinuities across fluid phases.
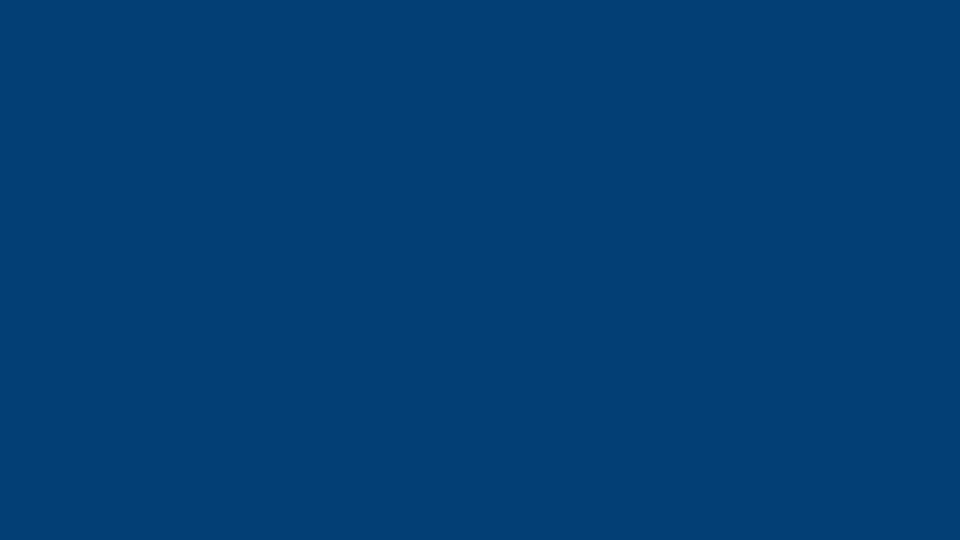
Drop breakup from a nozzle
Three-Dimensional Direct Numerical Simulation of Drop Coming out from a Nozzle.
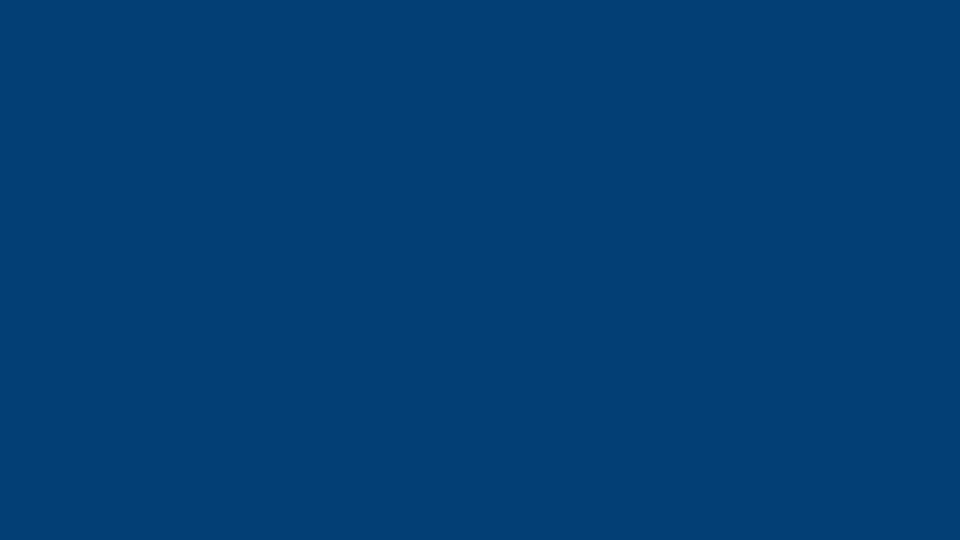
3D DNS falling film annular flow Re~600
3D DNS falling film annular flow Re~600
Theme membership
Theme affiliates: