Laboratory Astrophysics
High Energy Density facilties, such as high powered lasers and pulsed power generators like MAGPIE, are capable of reaching the extreme conditions found in astrophysical objects such as supernovae, black hole accretion disks and solar flares. Through "dimensionless scaling", we can directly compare our measurements of mm-scale, ns-lifetime plasmas to observations of objects which evolve over temporal and spatial scales which are 15 to 20 orders of magnitude larger. One example is jets from young stars, with typical lengths of thousands of astronomical units and evolving in timescales of many years. In order for this scaling to be valid, both the laboratory and astrophysical jets must have similar dimensionless parameters such as the Mach number, Reynolds number, Peclet number and the cooling parameter, among others.
Laboratory astrophysics experiments present a unique approach to producing and accurately studying a number of astrophysical scenarios. Experimental data allows computer codes to be verified, and, in addition, they are a discovery tool providing insights into the behaviour of complex highly non-linear systems.nOn MAGPIE, we study a range of astrophysical systems, including jets from young stars, radiative shocks, magnetic reconnection, bow shocks and accretion disks.
Supersonic Plasma Jets - Francisco Suzuki-Vidal
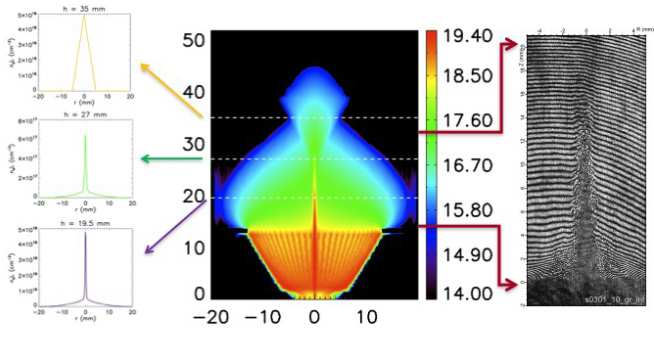
My experiments in MAGPIE look at the formation of supersonic plasma jets and their relevance to astrophysical jets, particularly those observed from protostars also known as Young Stellar Objects (YSOs). Some of my experiments have looked at jet launching and collimation by producing ‘magnetic tower jets’ using configurations such as radial wire arrays and radial foils. With the latter, it is possible to produce quasi-episodic magnetic tower outflows in a single experiment.
I am also interested in the formation of shocks in plasmas, which can be used to study terminal bow shocks from the interaction of a jet with the surrounding ambient medium, also known as Herbig-Haro objects. This can be done by injecting a neutral gas in the region above the foil or by looking at the head-on collision between two opposite radial foils. The formation of hydrodynamic instabilities is prevalent in these shocks.
Recently, I have been involved in new experiments on MAGPIE looking at the physics of differentially rotating plasmas which can be relevant to accretion disk physics and jet rotation.
Magnetic Reconnection and Magnetised Turbulence - Jack Hare
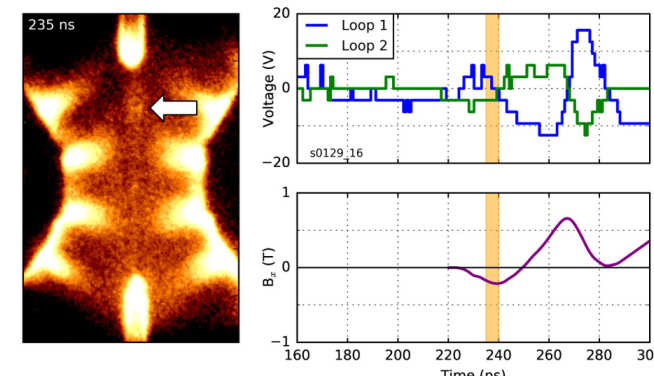
When oppositely directed magnetic field lines meet inside a plasma, they annihilate and 'reconnect', forming new field lines that connect different regions of plasma than the lines which formed them. Along with this reconfiguration, the energy stores in the magnetic field is released, causing the plasma to heat up and accelerate outwards at high velocities. This process is fundamental to understanding phenomena such as solar flares, the Aurora and exotic astrophysical phenomena such as magnetar flares and Gamma-ray bursts.
In MAGPIE, we collide fast moving jets of plasma which carry oppositely directed magnetic fields. These fields reconnect, and we observe how the magnetic field is destroyed, leading to plasma heating and the formation of "plasmoids", fast moving blobs of plasma that violently tear the reconnection process apart. These plasmoids cascade energy down to ever smaller length scales, and naturally lead to the development of turbulence within the plasma. Recently, I have been working on platforms for rapidly generating magnetised turbulence, and new diagnostics to study turbulence in unprecedented detail.
Rotating plasmas - Vicente Valenzuela-Villaseca
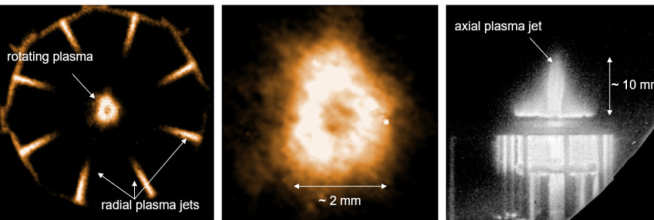
My research focuses on a new experimental platform producing differentially rotating plasmas on the MAGPIE generator. Rotating plasmas orbiting a central object (like a protostar or a blackhole) in the shape of accretion disks are common throughout the universe. The outstanding questions on this field regard the instabilities generated by the interplay of differential rotation and magnetic fields in these disks, which enhance the angular momentum transport driving the observed accretion rates. Furthermore, these astrophysical systems naturally drive jets that also evacuate angular momentum from the disk.
My experiments work on the basis of the oblique collision of 8 magnetised supersonic ‘radial’ plasma jets generated in the ablation of a wire array Z pinch. When these jets merge, a dense differentially rotating plasma column is form, effectively simulating an accretion disk. In addition, a highly collimated axial jet is accelerated out of the plane of rotation, similar to the astrophysical case. Through this experimental platform I can study the stability, angular momentum transport, jet launching and collimation mechanisms in a controlled and self-consistent laboratory environment.
Magnetized shocks and magnetic reconnection – Lee Suttle

My research is centred around the experimental study of magnetized plasma interactions. This incorporates the study of magnetic reconnection as well as magnetized shock formations and features. These phenomena are ubiquitous in both space and astrophysical environments, where fast outflows from stars and other energetic sources sweep extended magnetic field lines into the paths of various astrophysical objects and interstellar media. The presence of magnetic fields adds complexity to the structure of these interactions, for example adding preferential directions to processes such as thermal conduction, or affecting particle trajectories and plasma compressibility; ultimately dictating the global structure of the systems in which the interactions take place.
To create analogous systems in the laboratory we generate supersonic flows of plasma from an “exploding wire array” source. This plasma flow contains “frozen-in” magnetic field lines, which are carried by the flow as it propagates. Placing objects in the path of this flow or setting up collisions between oppositely directed flows, allows us to observe a wide range of interaction topologies, in which the magnetic flux undergoes effects such as shock compression and/or annihilation & reconnection. Our spatially and temporally resolved diagnostic suite provides detailed measurements of how the plasma parameters change through these interaction regions, giving us an insight into the particle trajectories and energy exchange mechanisms.
Magnetized shocks and Exploded Wires - Danny Russell
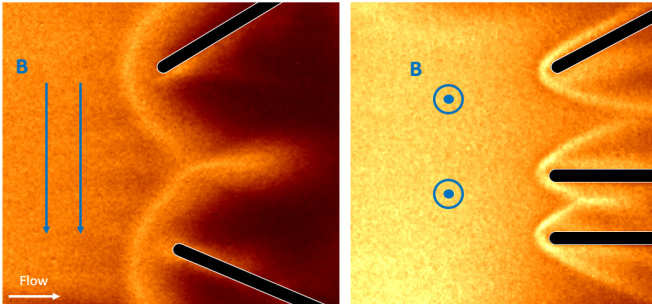
My work in MAGPIE focuses on magnetised shock reflections. Shock reflections are common in astrophysics, for example, in the internal structure of jets from young stars. To study shock reflections in the laboratory, we place multiple solid obstacles in the supersonic plasma flow from an exploding wire array. Magnetic field is “frozen-in” to the plasma and is strong enough to affect its dynamics. I study how the orientation of the magnetic field with respect to the shocks affects the properties of the shocks and the reflection topology.
Part of this research involves including exploded wires as obstacles. An exploded wire is produced by using a “pre-pulse” current to vaporise it. By using exploded wires as obstacles, we can study a broader range of shock behaviours.