Multidisciplinary research involves disciplines including magnetic design, MRI systems, control, signal processing, engineering analysis and design, shape modelling.
MRI research covers two main areas: novel MRI systems and interventional MRI. You can find out more about these areas below.
Geometric modelling and shape metrology
This research involves a wide range of methods for accurate modelling of free-form shapes, shape analysis, measurement and reconstruction.
Visit the Geometric modelling and manufacturing group website.
Novel MRI systems
Transverse field MRI for magic angle imaging
Many significant tissues containing collagen have short relaxation times which make them very hard to image using conventional MRI.
Changing the tissue orientation relative to the direction of the magnetic field can largely resolve this issue, and we have developed a magnet system which permits such changes.
This facility introduces a novel set of problems for imaging, data processing and magnet performance, which require physics and engineering research to resolve.
We propose to address the most important of these problems, arising from the orientation-dependent signal variation and the fact that the magnet needs to be moved relative to its surroundings.
Pairs of anatomical images obtained at different angle of the main field, arrows are used to mark tissues experiencing signal changes due to the magic angle effect.
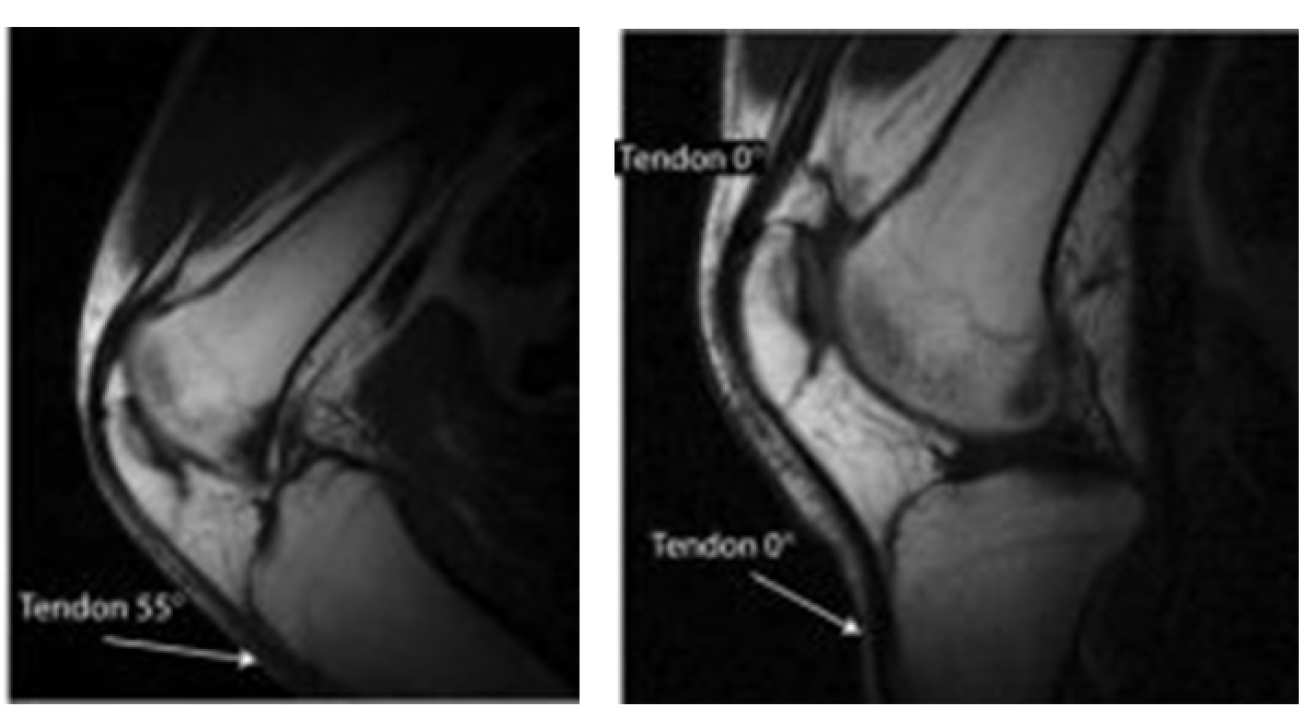

Possible magnet configurations
The magnet comprises a parallel pair of identical profiled arrays of permanent magnets backed by a flat steel yoke such that access in lateral directions is practical. The paper describes the detailed optimization procedure from a target 150 mm DSV to the achievement of a measured uniform field over a 130 mm DSV.
The Moveable MRI Magnet System
The assembly involves a 2 DOF mechanism, providing ‘roll’ and ‘yaw’ motion.
Compact Open MRI
Novel compact MRI has been designed for low cost, while offering unparalleled open patient access. This makes it suitable for a wide range of applications where the use of conventional closed-bore MRI has proved difficult, such as:
- Dealing with severely ill and/or claustrophobic patients for rapid diagnosiss
- Population screening such as for occlusive vascular disease
- Interventional MRI – wide range of procedures
Key features
- Novel asymmetric open magnet 0.5T 40cm gap, weight ~6 tonnes
- Use of MgB2 HTSC
Low cost design
- Single cryostat (bottom pole only)
- Permanent magnet arrays for field shaping
- ‘Dry’ magnet (no liquid He)
- Low power requirements: <10kVA using energy storage
‘Pod’ installation: prefabricated mobile or semi-permanent unit
- Low installation cost
- Integrated services –UPS, backup generator, cooling (closed circuit), air-conditioning
- Patient handling
- Suitable for mobile installations
Interventional MRI
MRI guided liver ablation
Treatment of otherwise inoperable liver tumours using Laser Ablation (LITT)
Aims
- Improved accuracy using MRI-assisted needle targeting, remote control
- Reduced setup time, overall procedure time
- MR thermometry using interstitial microcoils
- Early patient trial conducted successfully, further trials planned
Pneumatic positioning system
- Fully MR compatible, commercial actuators
- Compliance, friction, non-linear characteristics
- All control gear outside magnet room
- Long supply lines (9m)
- compliance, time delays
- Challenging non-linear control problems
- Sliding Mode Controller (SMC)
- Time Delay Control (TDC)
MRI-guided biliary endoscopy
- ERCP (Endoscopic Retrograde Cholangiopancreatography) is used to diagnose and treat biliary blockages and strictures
- High rate of catheterisation failure under fluoroscopy guidance
Project aims
- MR-compatible endoscope
- Remote-control operation inside MRI magnet
- High quality local imaging using interstitial coils
- Endoscope tip coil
- Catheter coil
Completed actuation system consists of three modules, independently driving 6 duodenoscope DOFs.
- Right hand module driving endoscope flexible section feed and twist
- Left hand module driving tip steering wheels and catheter deflection lever
- Catheter feed module feeding catheter into endoscope
MRI-guided prostate biopsy
Unlike previously proposed robotic devices,our system has no cables, sensors and motors inside the magnet bore, therefore it is inherently MRI safe. Probe is tracked directly in real-time by the MRI scanner, using semi-active markers with subpixel accuracy.
The fast tracking method can be interleaved with intraoperative image acquisition to aid accurate navigation. No coordinate transformation calculations required – degrees of freedom of the remote centre manipulator correspond to intuitive probe movement during procedure. No robot registration to the scanner is needed – registration errors eliminated – enables rapid setup even with the patient already in the scanner.
Design of the remote-centre manipulator (4 DOF) with the biopsy gun.
Owing to the direct tracking of the probe using MR sequences, there are no special registration nor calibration requirements at the start of the procedure.
Schematic layout during MRI guided biopsy procedure.
Manipulator configuration was designed to match the natural movements of the probe during the procedure.
Biopsy probe is inserted after the probe is aligned.
The patient remains in the scanner throughout the procedure.
Prototype in use in hospital MRI during trials with a realistic pelvic phantom. (right)
Interventional MR image feedback. Oblique sections through 3D image volume are automatically updated using probe position tracking and updated in real time. Synthetic image of the probe and guidance information are superimposed on MR image data. (below).
Real-time tool tracking for interventional MRI
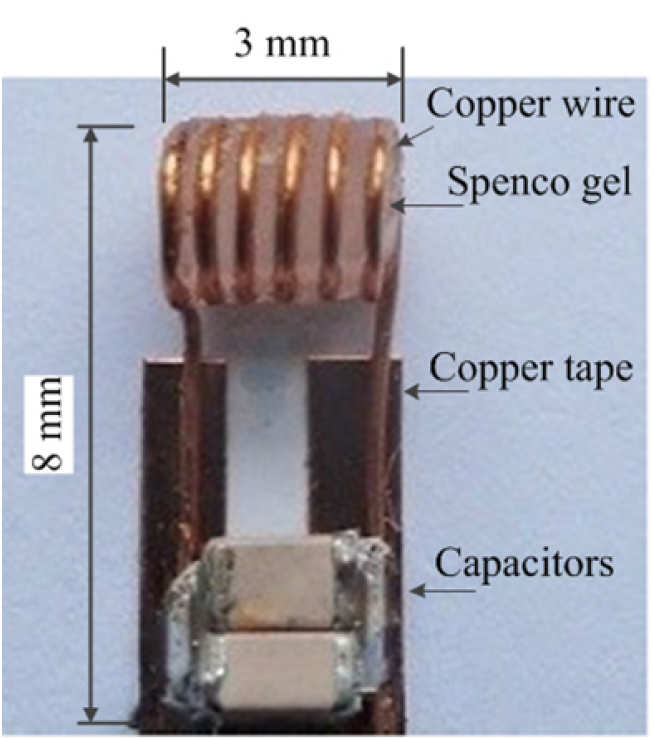
Interventional MRI procedures are often dependent on the localisation of objects or devices inside the scanner and many tracking schemes have been developed using either passive markers or micro-coil fiducials.
Tracking method
- Tracking of N fiducials based on a set of pre-determined 1D projections in space.
- The method computes the 3D position of N fiducial markers directly from 1D projections instead of deriving the 3D information from two orthogonal scan planes
The algorithm for N=2 markers
3 = 8 intersection points are computed as candidates (a); by using a fourth projection 4 fictitious points are eliminated (b) and by using a fifth projection (c) only the true points are left.
- 1D FT of the signal per each projection
- Peaks identification along each projection
- For each projection line, N planes are defined: each plane is normal to the projection line at one of the N projection values.
- A set of 3 reference planes is selected, according to descending order of maxima of minimum distances between the peaks in the corresponding projection.
- N3 intersection points are computed.
- Removal of (N3-N) points using non-reference directions.
Achievement
- Robust and accurate real-time tracking of devices within the scanner for MR image-guided interventional procedures
- Subpixel accuracy
- Sustainable refresh rate of 10 Hz for 3D position
x | y | z | 3D | |
---|---|---|---|---|
Standard deviation (mm) | 0.024 | 0.040 | 0.058 | 0.037 |
Maximum error (mm) | 0.105 | 0.090 | 0.148 | 0.208 |
Positional error in marker tracking (pixel size 1mm) |
People
Enrico Franco, Djordje Brujic, John McGinley, Quentin Herreros, Karyn Chappell
Collaborators
Ian Young, Richard Syms, Donald McRobbie, Justin Cobb, Wady Gedroyc, Nandita DeSouza, Simon Taylor-Robinson
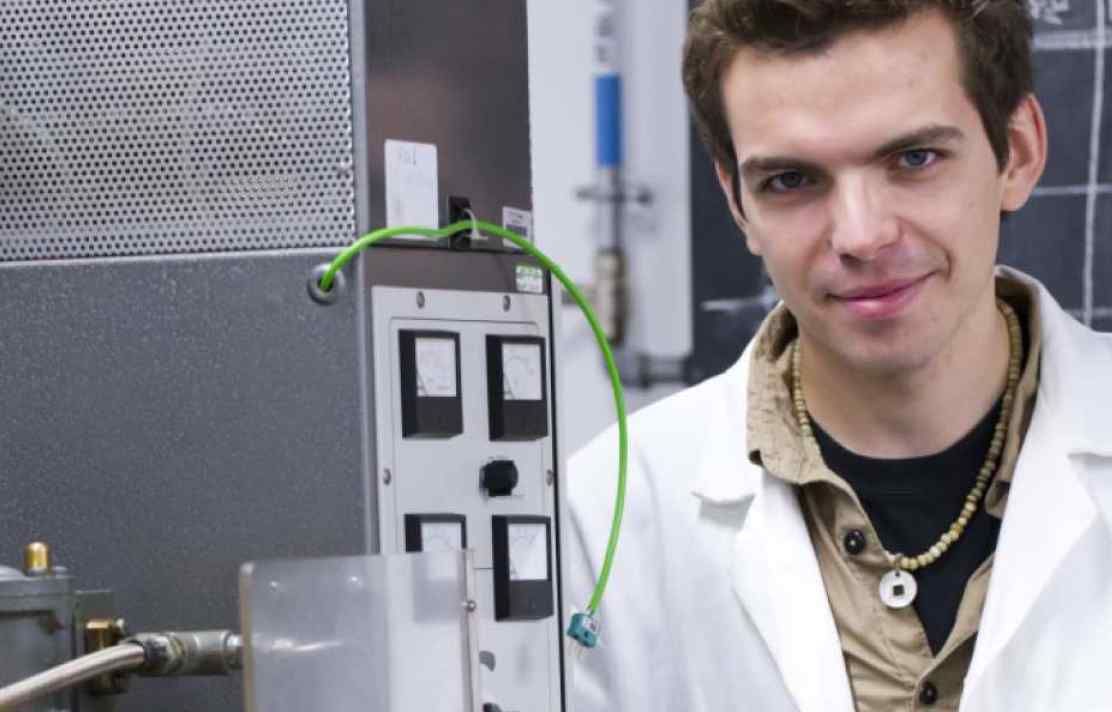
PhD study
Early Career Research Fellowships
Find out more about applying for early career fellowships in our department