Lithium-ion batteries
Lithium-ion batteries are essential components in a number of established and emerging applications including: consumer electronics, electric vehicles and grid scale energy storage. However, despite their now widespread use, their performance, lifetime and cost still needs to be improved. The ESE group works at a range of multi-disciplinary length scales to solve these problems with activities including: development of new materials, characterisation of these materials, modelling of their performance, thermal management of cells, development of control systems, analysis of battery pack designs, diagnostic techniques and techno-economic analysis.
Current projects
Machine learning based data fusion for advanced materials characterisation
Lead researchers: Dr Sam Cooper
PI’s: Dr Sam Cooper, Phil Withers (U. of Manchester), Jacqui Cole (U. of Cambridge)
Funding:
The performance of many devices is closely related to the geometry of the multiphase materials that they are made from (e.g. porous battery electrodes). Simulations can be used to better understand these relationships; however, they require the materials to be imaged in order to define an accurate simulation domain for meshing. In such cases microstructural data must:
- Be 3D.
- Be of high enough resolution to capture the key details.
- Have a large enough field of view to be representative of the material.
- Confidently differentiate the material phases present.
It is rare for data from a single imaging technique to satisfy all these requirements, meaning that simulations often lack vital information, undermining the value of any predictions. There is thus an urgent need for methods that can provide rich 3D information, in a fast and cost-effective manner. In this feasibility study, we will first characterise a diverse set of materials using three complementary techniques: X-ray computed tomography (XCT), focused ion beam scanning electron microscopy (FIB-SEM), and small angle scattering (SAS). Then we will use recently developed machine learning approaches to fuse the information from these approaches together. To this end we will:
- Collect at least five 3D microstructural, multi-modal and multiscale datasets acquired by a combination of nano XCT, large volume serial section 3D (laser or plasma beam) scanning and transmission electron microscopy. These data will be publicly archived (along with the materials themselves) to support the development of 3D microstructural modelling methods and protocols (Topic area: Materials Informatics).
- Demonstrate the feasibility of fusing information from distinct, but complementary, imaging techniques to accurately reconstruct multi-phase, high resolution, representative, 3D images and thus predict properties. Specifically, we use deep convolutional generative adversarial networks (GANs) to implement super-resolution, style transfer and dimensionality expansion (see. Figure 1). We will fuse complementary 2D and 3D images, combining different styles of information (morphological, as well as EBSD and EDX). Unlike the state-of-the-art, our approach does not
Publications:
-
Dahari A, Kench S, Squires I, Cooper S J, 2022, Fusion of Complementary 2D and 3D Mesostructural Datasets Using Generative Adversarial Networks, Advanced Energy Materials, 2202407.
SEATBELT
Lead researchers: Didier Devaux, CNRS
PI’s: Dr Sam Cooper and Dr Ainara Aguadero
Funding:
- Horizon Europe (Innovate UK backed)
Electric vehicles are powered by batteries, which are the most important part. But the demand for electric vehicles is increasing so fast that it will soon outpace battery cell production. The EU-funded SEATBELT project will help to pave the road towards a cost-effective, robust all-solid-state lithium battery comprising sustainable materials by 2026. Specifically, it will achieve the first technological milestone of developing a battery cell that meets the needs of the electric vehicle industry. The low cost cell will be safe by design with sustainable and recyclable materials, reaching high energy densities and long cyclability in line with the 2030 EU targets. The project will be the starting point of the first EU all-solid-state battery value chain.
Facilities:
-
Hi-Five
Collaborators:
- Universite Savoie Mont Blanc, France
- Polykey Polymers Sl, Spain
- Blue Solutions, France
- Basque Center For Macromolecular Design And Engineering Polymat Fundazioa, Spain
Publications:
-
tbc
Modelling of Electrochemical–Thermal-Mechanical Degradation in Lithium-Ion Batteries
Lead researchers: Dr Simon O’Kane, Dr Niall Kirkaldy, Dr Shen Li, Mr Jason Jiang, Dr Mingzhao Zhuo
PI’s: Dr Gregory Offer, Dr Monica Marinescu, Dr Billy Wu and Dr Huizhi Wang
Funding:
- EPSRC Faraday Institution, Multi-Scale Modelling project (EP/S003053/1, grant number FIRG003)
- Innovate UK BATMAN (BAttery Thermal MANagement and Diagnostics for Heavy Duty Applications, 104180), collaborative with Perkins Engines and AVID Technologies. 2018-2021.
- Innovate UK WIZer Batteries project (104427), collaborative with Williams Advanced Engineering, Silver Power Systems, and Codeplay. 2019-2022.
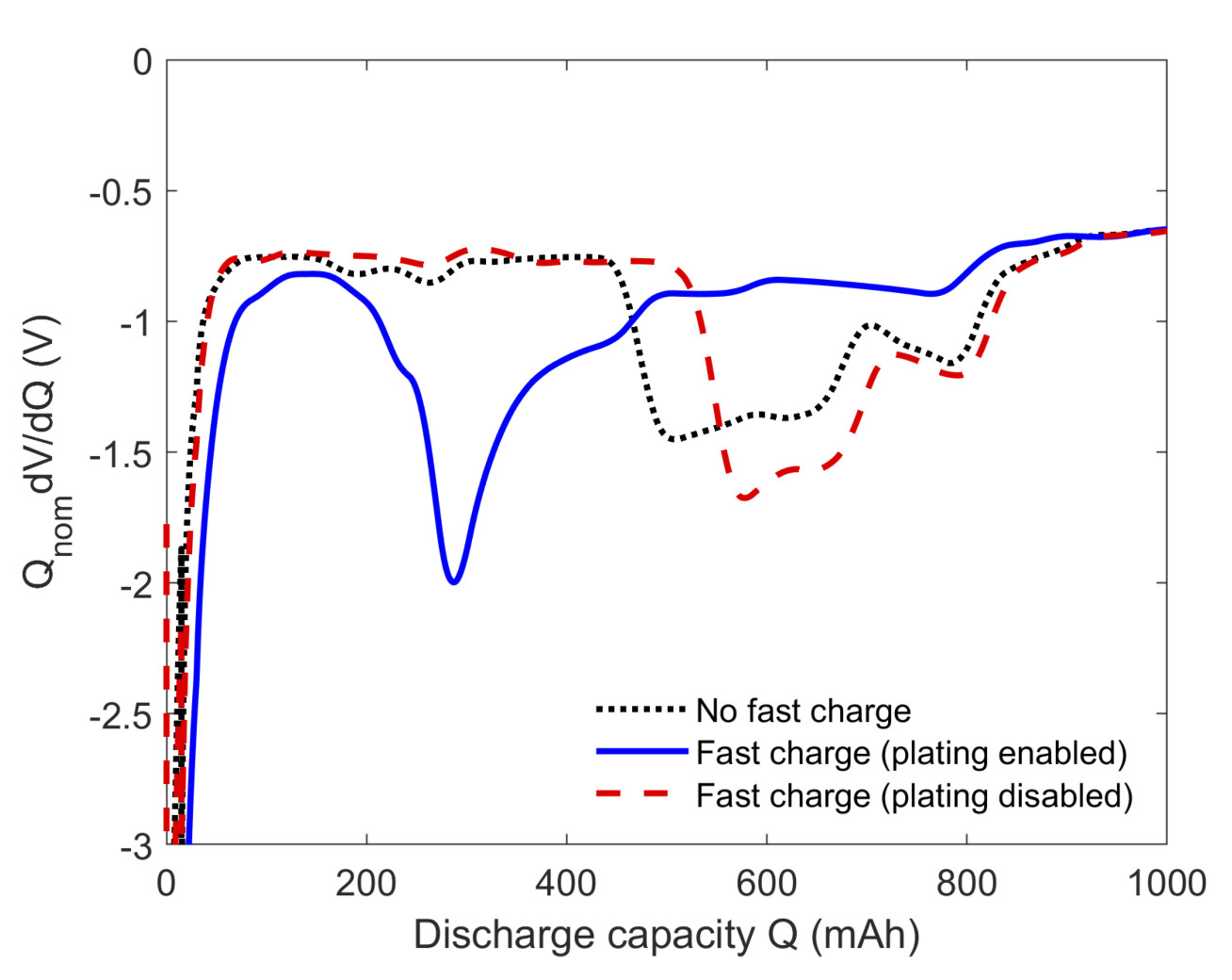
Understanding battery degradation is critical for cost-effective decarbonisation of both energy grids and transport. However, battery degradation is often presented as complicated and difficult to understand.
Battery degradation has many different causes including unwanted electrochemical side reactions, self-heating and mechanical wear and tear. Being able to detect and prevent degradation requires a greater physical understanding of each mechanism than we currently have. One way of gaining this understanding is physics-informed computer modelling.
One of the main active areas of research of the ESE group is to develop and implement high fidelity physics based and equivalent circuit network models to predict and investigate the effects of degradation on LI-ion batteries. Our group works with and contributes to the open-source modelling platform PyBaMM (Python Battery Mathematical Modelling), which was created by the University of Oxford Mathematical Institute.
The modelling work is accompanied with data provided by accurate and extensive battery tests performed in our laboratories, using custom made battery rigs and the best state of the art battery cyclers.
Publications:
-
O’Kane SEJ, Campbell ID, Marzook WJ, Offer GJ and Marinescu M, 2020, Physical Origin of the Differential Voltage Minimum Associated with Lithium Plating in Li-Ion batteries, Journal of the Electrochemical Society, Vol: 167, Pages: 090540.
Ghosh A, Foster JM, Offer G, Marinescu M, 2021, A Shrinking-Core Model for the Degradation of High-Nickel Cathodes (NMC811) in Li-Ion Batteries: Passivation Layer Growth and Oxygen Evolution, Journal of the Electrochemical Society, Vol: 168, Pages: 020509
-
Edge JS, O'Kane S, Prosser R, Kirkaldy ND, Patel AN, Hales A, Ghosh A, Ai W, Chen J, Yang J, Li S, Pang M-C, Bravo Diaz L, Tomaszewska A, Marzook MW, Radhakrishnan KN, Wang H, Patel Y, Wu B, Offer GJet al., 2021, Lithium ion battery degradation: what you need to know, Physical Chemistry Chemical Physics, Vol: 23, Pages: 8200-8221, ISSN: 1463-9076
-
Li R, O’Kane S, Marinescu M, Offer J, 2022, Modelling solvent consumption from SEI layer growth in lithium-ion batteries, Journal of the Electrochemical Society, Vol: 169, Pages: 060516.
Modelling Li-ion battery fast charging
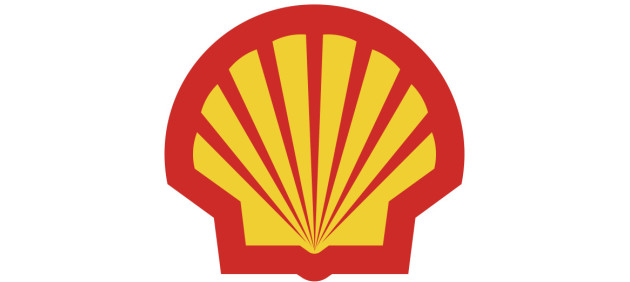
Lead researcher: Miss Anna Tomaszewska
PI’s: Dr Billy Wu and Dr Gregory Offer
Funding:
- Shell UK
Range anxiety is often quoted as a key barrier to the widespread adoption of electric vehicles. To compete with internal combustion engines, batteries must be capable of recharging to 80% state of charge in about 10 minutes. However, fast charging is known to result in accelerated capacity and power fade particularly in high energy cells, where the transport of lithium ions is hindered by thicker electrodes. When the rate of current supply outpaces the rate of ion diffusion, lithium may begin depositing on the anode instead of intercalating into it, leading to the loss of lithium inventory, local electrode deactivation, and internal short circuits in extreme cases.
The project focuses on model-based approaches to optimize the fast charging performance of Li-ion batteries. The first outcome was a comprehensive review of the existing fast charging strategies from the material to cell and pack level. Although many promising material improvements were identified, engineering solutions such as current profile and thermal management optimization were found to be achievable over much shorter timescales and at a lower cost. The project aims to address two of the key knowledge gaps identified in the review:
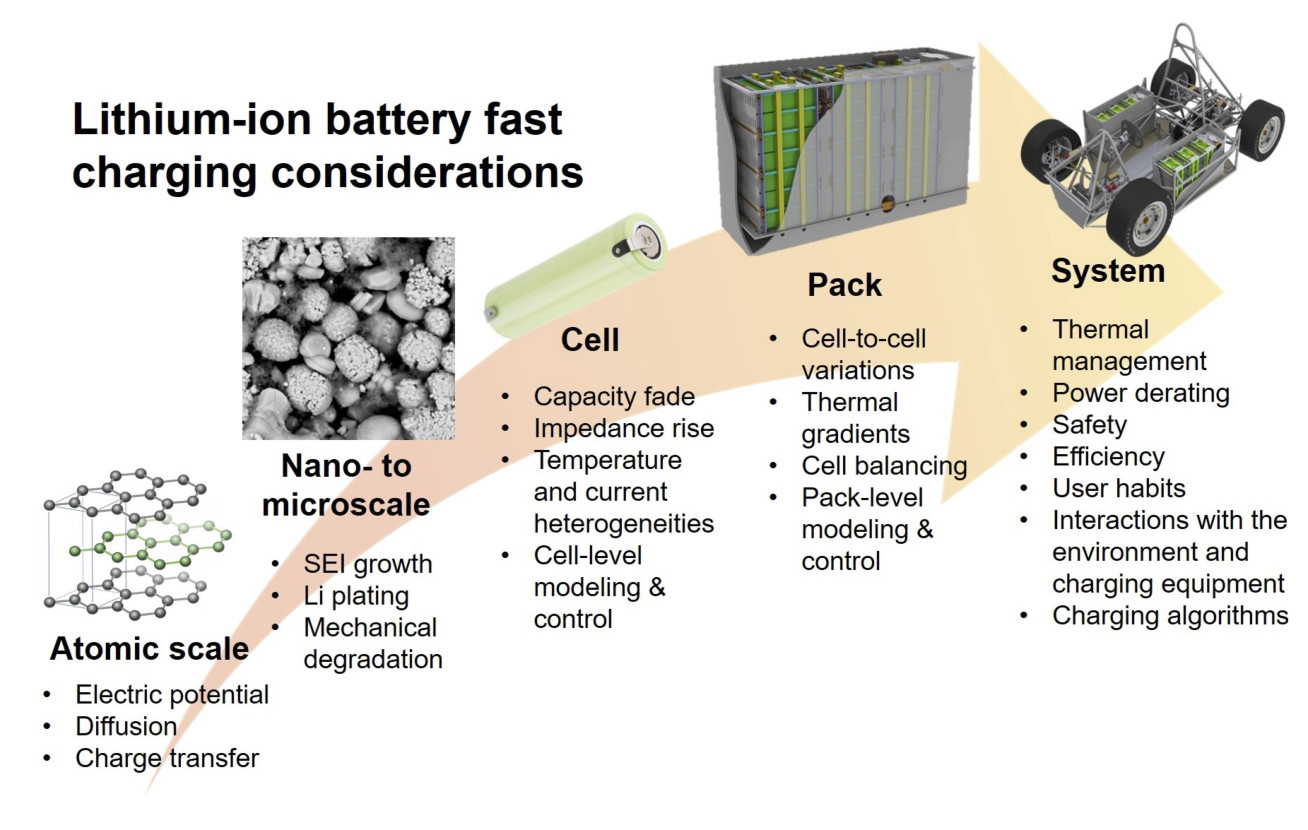
- Out of the many fast charging protocols proposed in the literature, very few have been evaluated at temperatures other than room temperature or for more than one cell type.
- The understanding of fast charging performance under realistic thermal conditions is very limited. Most studies have been conducted under isothermal conditions or in a climate chamber, while individual cells in battery packs can experience significant transient and spatial thermal gradients.
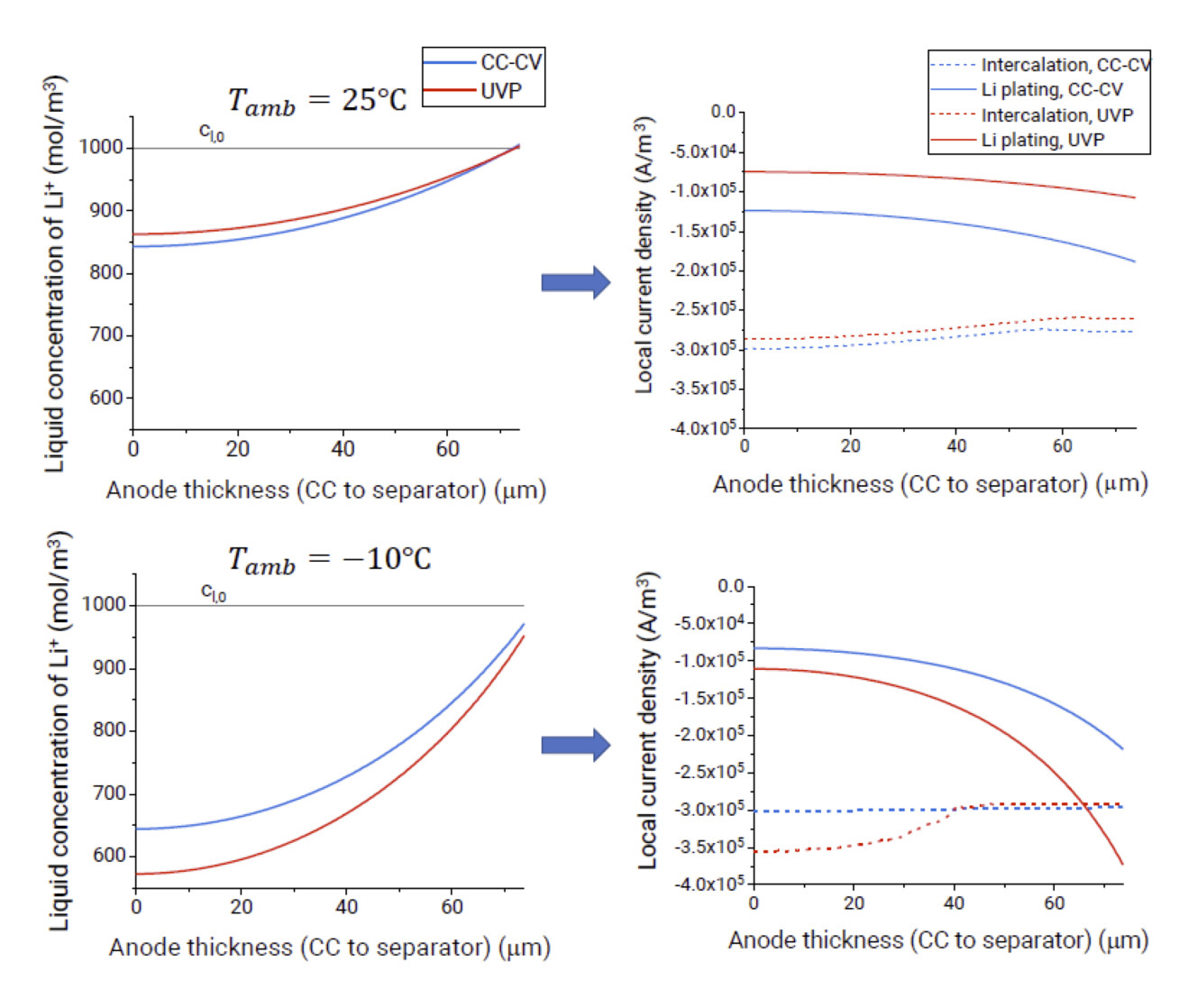
Initial work on physics-based modelling of fast charging protocols has shown that charging profiles can perform differently under different environmental conditions or for different cells. For example, a charging protocol known as the Universal Voltage Protocol (UVP), previously demonstrated to significantly reduce degradation when used at room temperature, has been shown to be potentially more damaging than standard constant current – constant voltage (CC-CV) charging at subzero temperatures in high energy cells due to electrolyte depletion effects.
Recently, the model has been extended to 2D and 3D to investigate the effects of thermal gradients that typically arise in battery packs on the lithium plating behaviour, including both the plating distribution and severity compared to a thermally uniform cell. Experimental validation is ongoing. The findings of the project will hopefully inform the selection of appropriate fast charging strategies based on the ambient conditions, thermal management layout, and cell type.
Publications:
- Tomaszewska A, Chu Z, Feng X, O’Kane S, Liu X, Chen J, Ji C, Endler E, Li R, Liu L, Li Y, Zheng S, Vetterlein S, Gao M, Du J, Parkes M, Ouyang M, Marinescu M, Offer G, Wu B, 2019, Lithium-ion battery fast charging: A review, eTransportation, Vol: 1, Page: 100011.
New electrolytes for lithium batteries
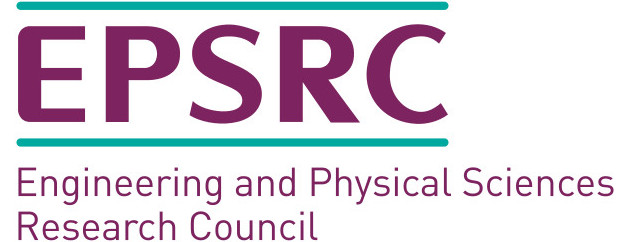
Lead researcher: Dr Yan Zhao
PI: Dr Huizhi Wang
Funding:
- EPSRC UK grant, Smart Microfluidics Towards Low-Cost High-Performance Li-Ion Batteries (EP/S000933/1)
Metallic Li anode can react with carbonate electrolytes, which can lead to continuous side reactions between Li and electrolytes during repeated Li plating/stripping, and eventually cause low Li Coulombic efficiency and poor cycle life. Ether-based electrolytes are regarded as an excellent candidate because of their high reductive stability with Li. However, the oxidation instability of ethers beyond 4.0V have limited their application in practical high-voltage lithium metal batteries. This research is to develop an ideal electrolyte system for high-voltage metal Li batteries by investigating novel Li salts or electrolyte additives.
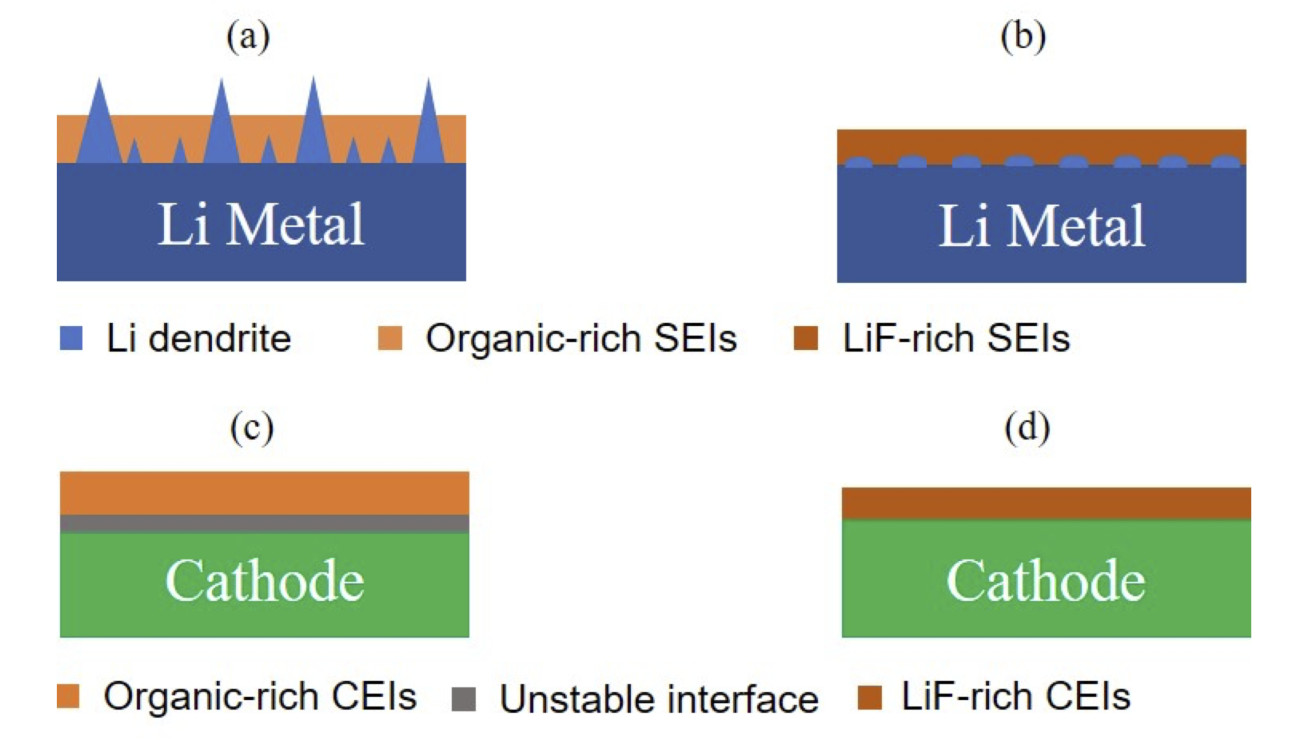
The latest common sense about electrolytes is to develop high-concentration electrolytes, which can effectively enhance their oxidation stabilities and the cycle life of high-voltage electrodes. However, the high concentration electrolytes are facing several challenges such as high viscosity and subsequent low ionic conductivity as well as high cost. The latest finding is that some fluorinated solvents or additives can contribute to the formation of stable LiF-rich SEI on metal Li surface or CEI on high-voltage cathode surface (Figure 1), which can improve the stable cycling of high-voltage Li metal batteries.
X-ray photoelectron spectroscopy (XPS) is conducted to analyse the surface composition of SEIs or CEIs. Scanning electron microscope (SEM) and transmission electron microscope (TEM) are used to characterize the morphology and structure of the samples. The electrochemical properties are completed by the electrochemical workstation and the cycling data of the cells are collected on Land battery testers.
Collaborators:
- ESE group - Department of Mechanical Engineering: Mr Yang Jiang
Publications:
-
Pan W, Wang Y, Zhao X, Zhao Y, Liu Y, Xuan J, Wang H, Leung DYC, 2021, High-Performance Aqueous Na–Zn Hybrid Ion Battery Boosted by “Water-In-Gel” Electrolyte, Advanced Functional Material, pages: 2008783
Silicon-based lithium ion batteries

Lead researcher: Mr Jason Jiang
PI’s: Dr Huizhi Wang and Dr Gregory Offer
Funding:
- UK Engineering and Physical Sciences Research Council (EPSRC) (via grant EP/S000933/1)
- Innovate UK WIZer project (TS/S005811/1)
- Faraday Institution Multiscale Modeling project (EP/S003053/1, via grant FIRG003)
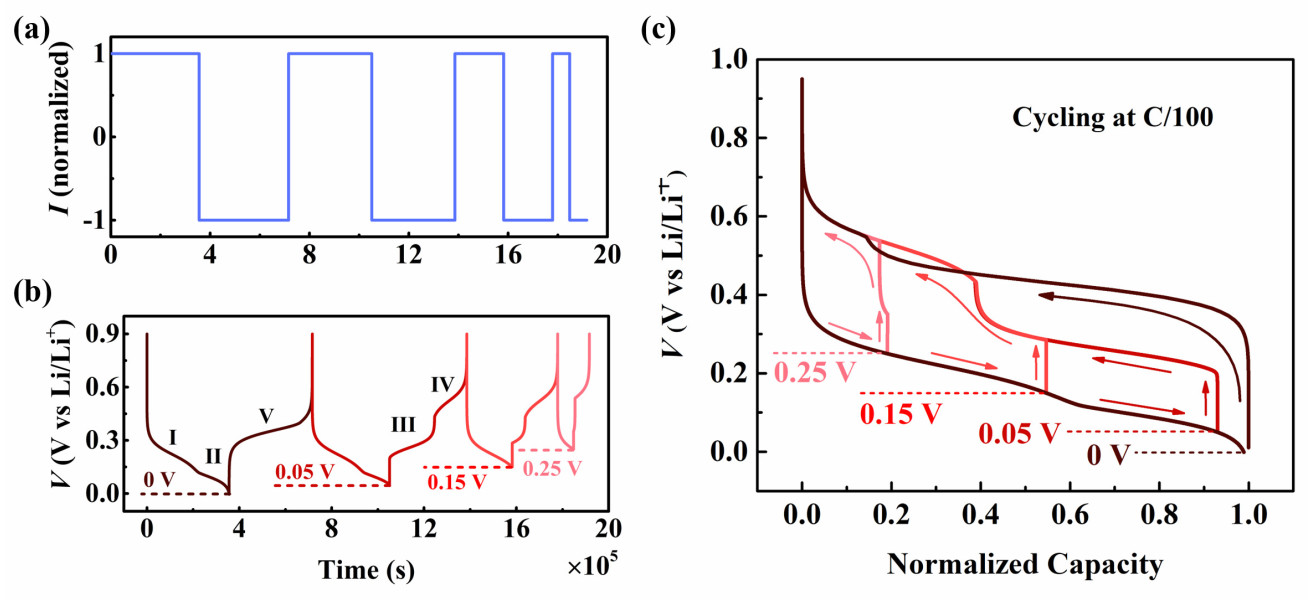
Silicon is one of the most promising anode materials to be used in the next generation high energy density lithium-ion batteries (LIBs) due to its large specific capacity, abundant reserves and little toxicity. As a kind of alloying material, silicon exhibits unique electrochemical behaviours compared to the conventional anode material graphite/carbon, particularly the well-known path-dependent voltage hysteresis observed during battery cycling. These unique electrochemical behaviours of silicon are highly correlated to the electrochemical phase transformations, crystallization and amorphization of Li-silicon phases. However, these important behaviours of silicon lack a comprehensive understanding of the existing theoretical studies. This project specifically focuses on the modelling study of the electrochemical features of silicon-based Li-ion batteries. The model can be a necessary tool for future design and development of high-energy-density, longer-life silicon-based LIBs.
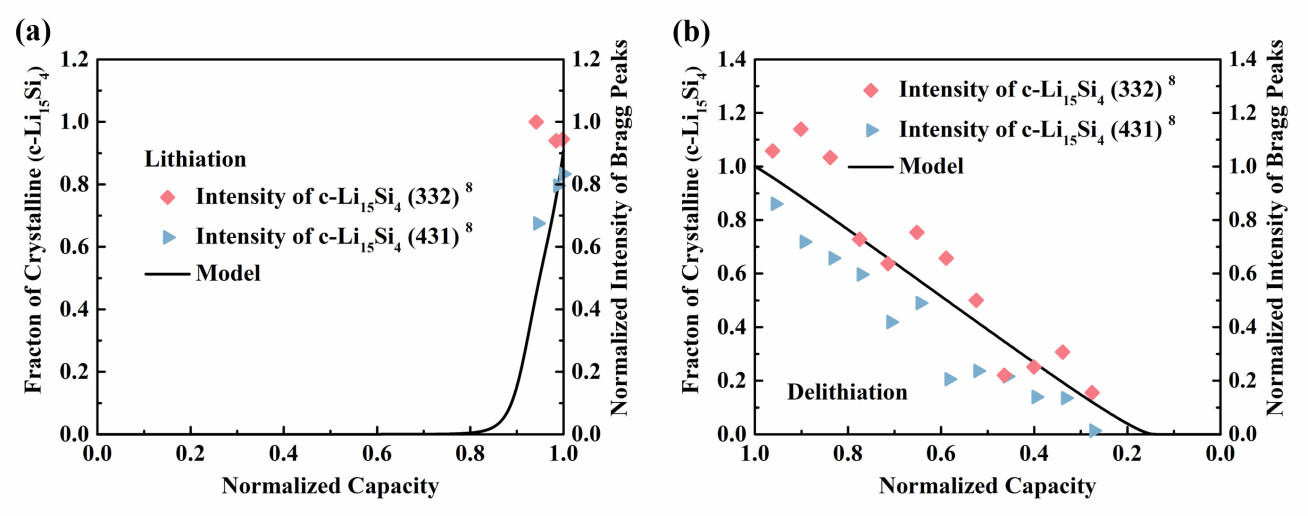
This model considers the multi-step phase transformations, crystallization and amorphization of different lithium-silicon phases during cycling while being able to capture the unique voltage hysteresis under different lithiation depths. During the first cycle (Figure 1(b)) when the voltage falls to 0 V, the silicon electrode undergoes two-phase transformation stages I and II. After the current reversal, the voltage curve presents a distinct plateau which suggests the amorphization process V. During the following cycles when the lower voltage limits rise above 0.05 V, the lower voltage branch follows the same trace as that in the first cycle, while the higher voltage branch turns to be a sigmoidal structure, which implies the two-phase transformation stages III and IV.
This developed model can also retrieve the sudden formation of the crystalline Li-Si phase during charge and its gradual disappearance during discharge (Figure 2).
Collaborators:
- ESE group - Department of Mechanical Engineering: Dr Monica Marinescu
- Department of Mechanical Engineering: Dr Jun Jiang
Publications:
-
Jiang Y, Offer GJ, Jiang J, Marinescu M, Wang H, 2020, Voltage Hysteresis Model for Silicon Electrodes for Lithium Ion Batteries, Including Multi-Step Phase Transformations, Crystallization and Amorphization, Journal of the Electrochemical Society, Accepted Manuscript.
Cell Design Optimisation Modelling
Lead researchers: Dr Tao Zhu, Dr Carlos Garcia-Gonzalez, Dr Han Yuan, Dr Mohammad Samieian
PIs: Dr Gregory Offer, Dr Yatish Patel, Dr Monica Marinescu
Funding:
- Faraday TOPBAT (Thermally Optimised Batteries, FIRG023). 2020-2021
- Innovate UK Power cell Up-Scaling project (10007479), collaborative with AMTE power and the UK-Battery Industrialisation Centre (UKBIC). 2021-2022
- Innovate UK GENESIS (Generating Energetic Novel cells and Systems Inspired by Software, 10007488) project, collaborative with BritishVolt, Johnson Matthey and Entek ltd. 2021-2022
Lithium-ion cells generate a considerable amount of heat during operation, particularly for demanding applications such as hybrid and electric vehicles. Cell behaviour is strongly influenced by temperature, since it affects impedance exponentially. Therefore, a badly designed cell from a thermal perspective will lead to reduced power, less usable capacity, and ultimately reduced energy density and life at pack level.
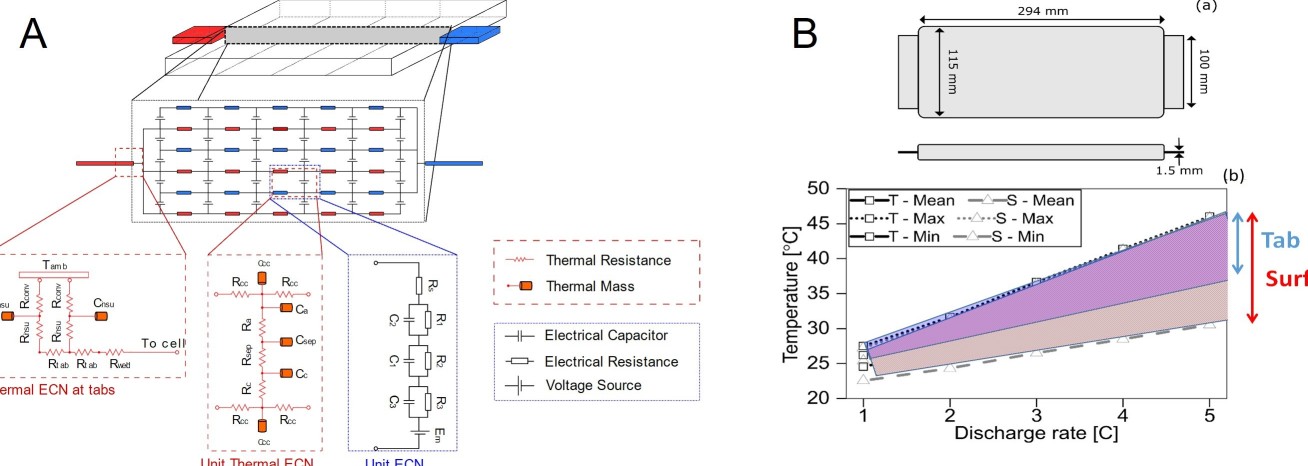
The ESE group team at Imperial College London have been working on understanding cell thermal gradients and overall thermal performance of cells for different cooling strategies. With the aim of improving cell performance and cycle life, they have developed a new metric, the Cell Cooling Coefficient, CCC (W K-1), to evaluate the thermal performance of lithium-ion cells independently of their chemistry, form factor or manufacturer. The CCC, which can be measured empirically via standardized experiments, quantifies the heat rejection capability of a cell for a particular thermal management method, and can also be used to evaluate cell heat generation for a given operating condition. The research shows how this understanding can be used to optimise the design of cells.
Focus of current research projects in the ESE group is to deliver a breakthrough in cell design thorough modelling approach, optimising thermal performance by opening up present thermal bottlenecks in cells. The models will minimise design iterations, exploring the trade-offs between energy, power, heat generation and rejection, for high power applications under tab cooling.
Publications:
- Offer G, Patel Y, Hales A, Bravo Diaz L, Marzook M et al., 2020, Cool metric for lithium-ion batteries could spur progress, Nature, Vol: 582, Pages: 485-487, ISSN: 0028-0836
- Hales A, Prosser R, Diaz LB, et al., 2020, The Cell Cooling Coefficient as a design tool to optimise thermal management of lithium-ion cells in battery packs, Etransportation, Vol:6, ISSN:2590-1168
- Hales A, Marzook MW, Bravo Diaz L, Patel Y, Offer G et al., 2020, The surface cell cooling coefficient: a standard to define heat rejection from lithium ion battery pouch cells, Journal of The Electrochemical Society, Vol: 167, ISSN: 0013-4651
- Hales A, Diaz LB, Marzook MW, Zhao Y, Patel Y, Offer G et al., 2019, The cell cooling coefficient: A standard to define heatrejection from lithium-ion batteries, Journal of The Electrochemical Society, Vol: 166, Pages: A2383-A2395, ISSN: 0013-4651
Past projects
Electrode Engineering for Next Generation Lithium-ion Batteries
Lead researcher: Ridwanur Chowdhury
PI: Prof Nigel Brandon
Funding:
- EPSRC UK grant, Enabling next-generation lithium batteries (EP/M009521/1)
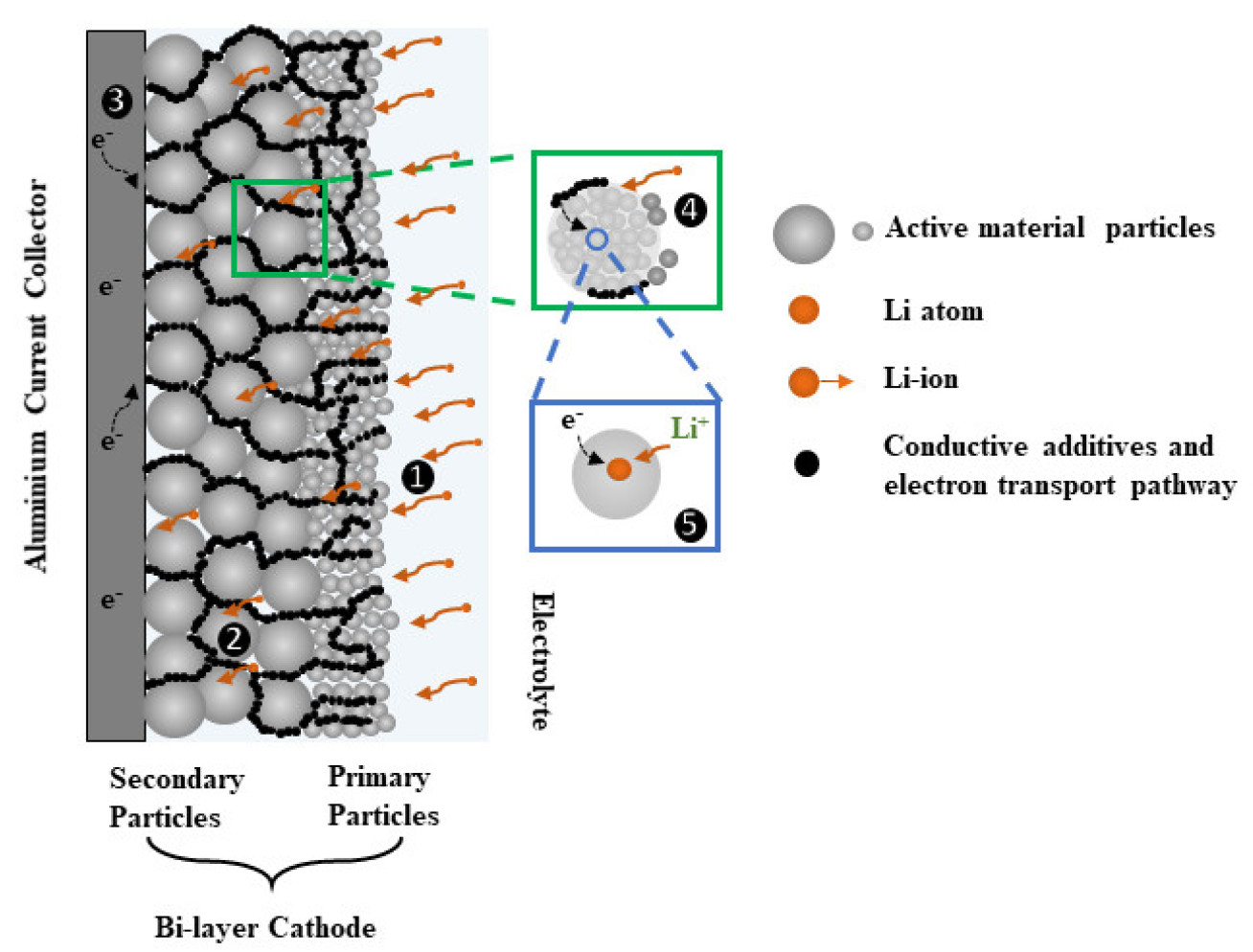
The need for the development of secondary lithium-ion batteries (LIB) with high power and high energy density is imperative for the advancement of portable devices, electric vehicles (EV), and integrated renewable energy system. Thick electrodes are attractive for high-energy LIB applications because of their potential capability to store high amounts of Li+with increasing amounts of active materials. However, as the electrode thickness increases, mass transport limitations in both solid and liquid phase become more important impeding the power delivery.
We are currently investigating thick graded cathode (NMC) microstructures by varying both particle size and corresponding porosity through electrode thickness direction. On the one side, particle sizes directly affect the mass transport in solid-phase through diffusion length, and on the other side, porosity affects the effective conductivity and diffusivity through tortuosity. Hence, the project focuses on designing and engineering thick graded microstructures for energy-power application through experimentation, advanced characterization (FIB, XCT) and simulation.
Collaborators:
- University of Twente: Dr Aayan Banerjee, Catalytic Processes and Materials, Faculty of Science and Technology.
Publications:
-
Chowdhury R, Banerjee A, Zhao Y, Liu X, Brandon N, 2021, Simulation of Bi-layer Cathode Materials with Experimentally Validated Parameters to Improve Ion Diffusion and Discharge Capacity, Sustainable Energy Fuels
-
Chowdhury R, Zhao Y, Xia Y, Ouyang M, Brandon N, Banarjee A, 2021, Revisiting the Promise of Bi-layer Graded Cathodes for Improved Li-ion Battery Performance, Sustainable Energy & Fuels
Battery pack modelling
Lead researchers: Mr Max P Naylor Marlow and Dr Weilong Ai
PI’s: Dr Billy Wu
Funding:
- EPSRC Faraday Institution Multi-Scale Modelling project (EP/S003053/1, grant number FIRG003)
- Imperial College London Doctoral Training Partnership (EP/N509486/1 project number 1854850)
The performance of lithium-ion battery packs are often extrapolated from single cell performance however uneven currents in parallel strings due to cell-to-cell variations, thermal gradients and/or cell interconnects can reduce the overall performance of a large scale lithium-ion battery pack. This project is to investigate the performance implications caused by those factors by experiments and simulations:

Experimental: Experimental work includes parameterisation and validation of models at the pack level, using our in-house custom pack test bench (Figure 1) to evaluate the behaviour of parallel cell strings under applied thermal conditions
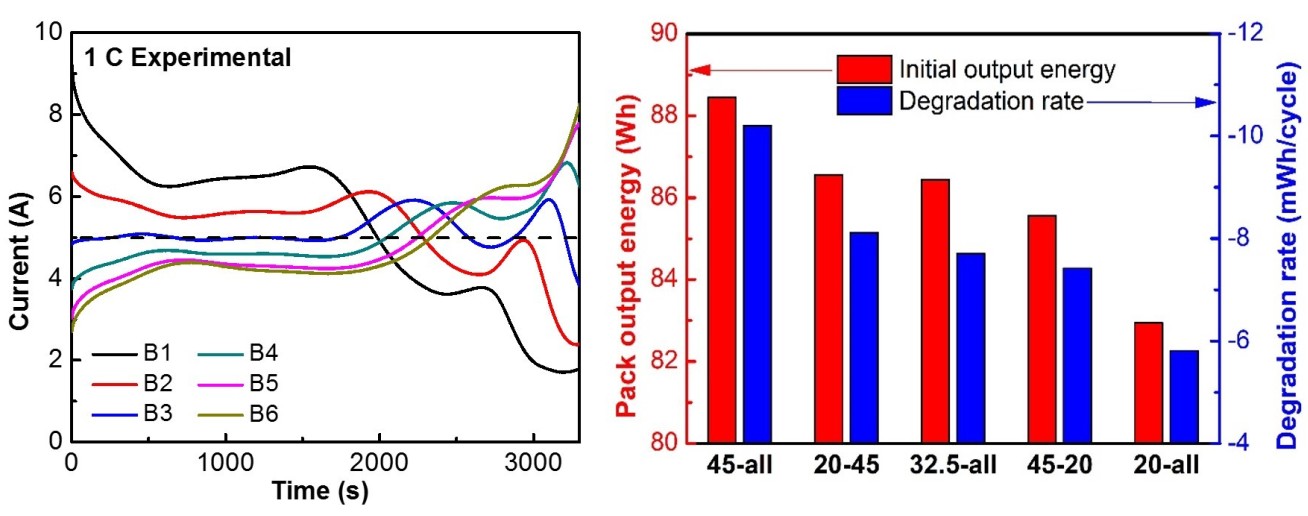
Theoretical: Thermally coupled single particle models are developed to analyse the performance of battery packs, with considering the growth of solid electrolyte interphase layers. The output energy and capacity degradation of battery packs are studied under different operating conditions (Figure 2).
This work will provide insights for the designs of higher performance and longer lasting battery packs.
Collaborators:
- UCL - Dept of Chemical Engineering: Dr Thomas Tranter
Publications:
-
Liu X, Ai W, Marlow MN, Patel Y, Wu B, 2019, The effect of cell-to-cell variations and thermal gradients on the performance and degradation of lithium-ion battery packs, Applied Energy, Vol: 248, Pages: 489-499.
Modelling/experimental validation of mechanical effects in lithium-ion batteries
Lead researchers: Dr Weilong Ai and Dr Jingy Chen
PI’s: Dr Billy Wu and Dr Gregory Offer
Funding:
- EPSRC Faraday Institution Multi-Scale Modelling project (EP/S003053/1, grant number FIRG003)
- Innovate UK Battery Advances for Future Transport Applications (BAFTA) Project, collaborative R&D with Aston Martin Lagonda and Dukosi Limited.
Electrode materials experience large volume change during lithium (de)intercalation, and the resulting stress can cause cracking in electrode particles. Those cracks release new surface area to allow side reactions including solid electrolyte interphase growth and lithium plating, which accelerate the capacity degradation of lithium ion batteries. This project is to investigate the root causes of particle cracking and to develop models to improve battery performance by alleviating particle cracking issues.
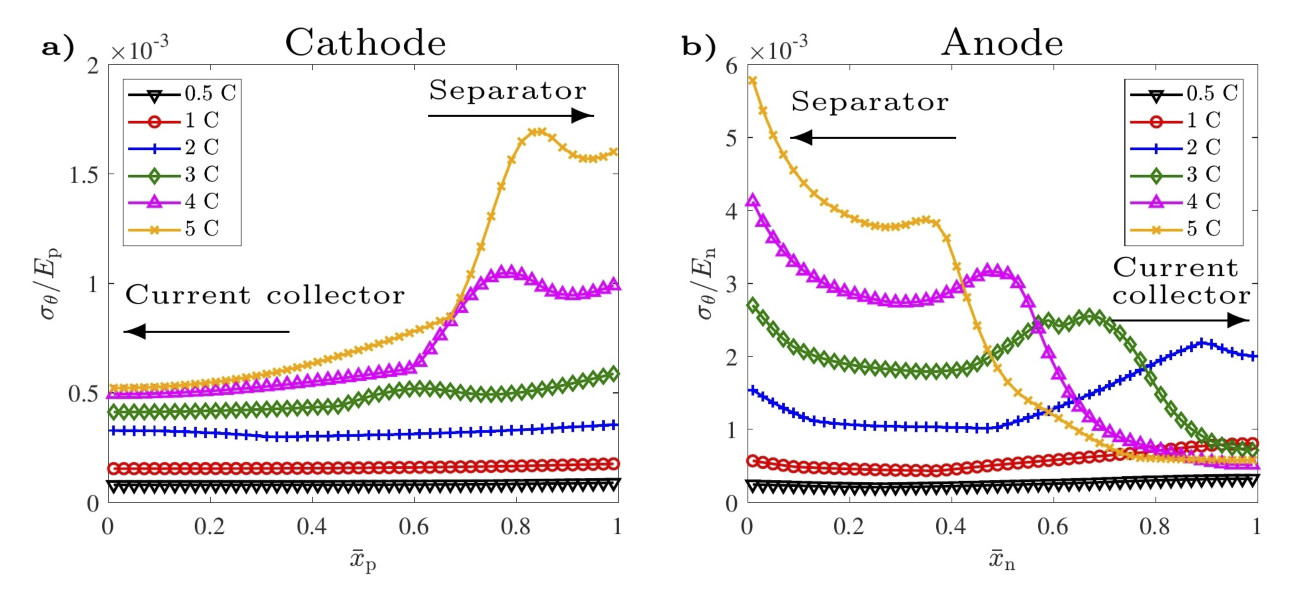
The latest findings include a developed stress model for batteries showing large stresses close to the separator for high C-rates (Figure 1) and nonlinear diffusivity of electrode materials causing severe particle cracking in a narrow range of state of charge. In line with the non-linear diffusivity, cell aging experiments are on-going to investigate the influence of SOC range, temperature and C-rate on the rate of degradation. Electron imaging on the electrode particles serves as direct evidence of the extent of cracking (Figure 2) in post-mortem analysis.
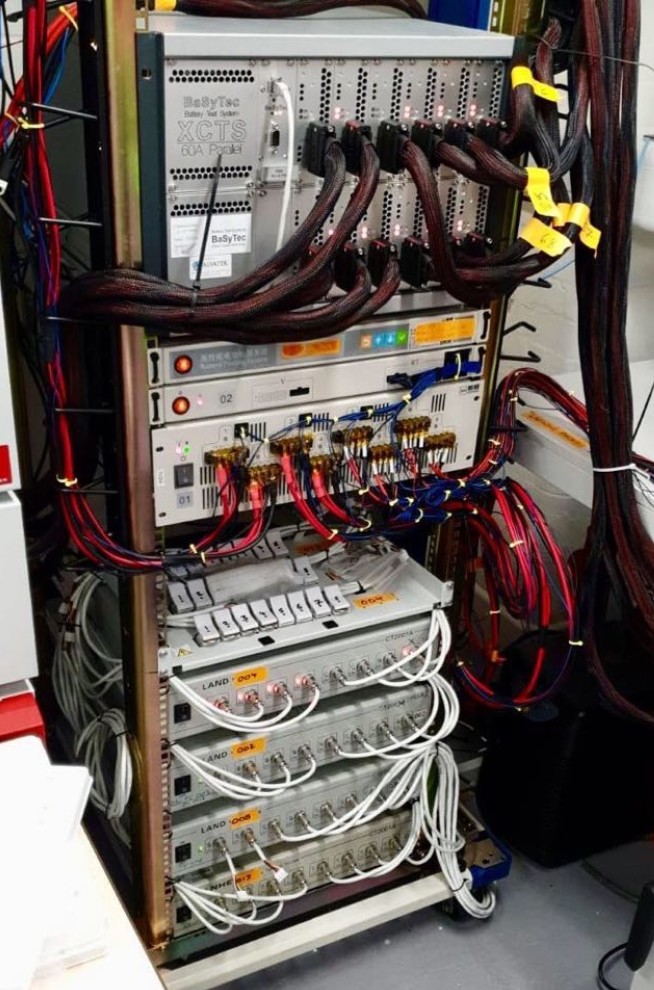
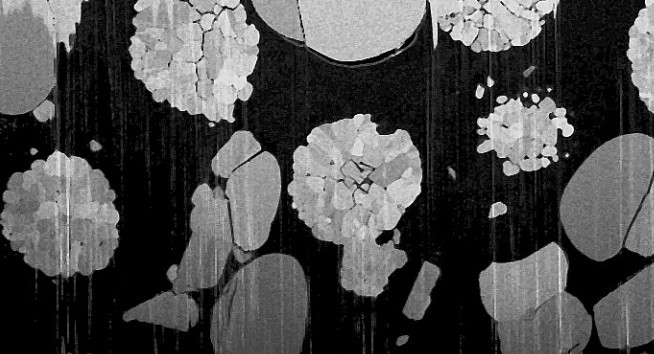
Non-invasive in-situ diagnostic techniques, incremental capacity analysis (ICA) and differential thermal voltammetry (DTV) are applied to quantify extent of cell degradation, which provides insights in real-time battery management for maximising both performance and longevity. Long-term test data are collected from in-house facility (Figure 3).
Collaborators:
- ESE group - Dyson School of Design Engineering: Mr Max Naylor Marlow
- Department of Civil and Environmental Engineering: Dr Emilio Martinez-Paneda
Publications:
-
Ai W, Kraft L, Sturm J, Jossen A, Wu B, 2019, Electrochemical Thermal-Mechanical Modelling of Stress Inhomogeneity in Lithium-Ion Pouch Cells, Journal of The Electrochemical Society, Vol: 167, Pages: 013512 - 013524
Conference presentations:
-
Ai W, Chen J, O’Connor TS, Marlow MN, Wu B, An electrochemo-mechanical coupled model for lithium ion batteries, Oxford Battery Modelling Symposium (OBMS 2020), March 16-17, 2020, Oxford, UK.
Recent publications, 2021 - to date
Ruan H, Varela Barreras J, Engstrom T, Merla Y, Millar R, Wu B, 2023, Lithium-ion battery lifetime extension: A review of derating methods, Journal of Power Sources, vol. 563, p. 232805.
Edge JS, Lander L, Brophy K, Hales A, 2022, The Value of Modelling for Battery Development and Use, Faraday Insights - Issue 15: December 2022, Institute for Molecular Science and Engineering Briefing Paper No. 8
Li R, O’Kane S, Marinescu M, Offer J, 2022, Modelling solvent consumption from SEI layer growth in lithium-ion batteries, Journal of the Electrochemical Society, Vol: 169, Pages: 060516.
Ruan H, Chen J, Ai W, W B, 2022, Generalised diagnostic framework for rapid battery degradation quantification with deep learning
Steinhardt M*, Barreras JV*, Ruan H*, Wu B, Offer GJ, Jossen A, 2021, Meta-analysis of experimental results for heat capacity and thermal conductivity in lithium-ion batteries: A critical review, Journal of Power Sources, (in press). *Joint first authorship.
Yang S, Zhou C, Wang Q, Chen B, Zhao Y, Guo B, Zhang Z, Gao X, Chowdhury R, Wang H, Lai C, Brandon NP, Wu B, Liu X, 2021, Highly-aligned Ultra-thick Gel-based Cathodes Unlocking Ultra-high Energy Density Batteries, Energy & Environmental Materials (accepted).
Xue S, Fu Y, Song Z, Chen S, Ji Y, Zhao Y, Wang H, Qian G, Yang L, Pan F, 2021, Coil-to-Stretch Transition of Binder Chains Enabled by “Nano-Combs” to Facilitate Highly Stable SiOx Anode, Energy & Environmental Materials (accepted).
Chowdhury R, Zhao Y, Xia Y, Ouyang M, Brandon N, Banarjee A, 2021, Revisiting the Promise of Bi-layer Graded Cathodes for Improved Li-ion Battery Performance, Sustainable Energy & Fuels
de Castro R, Pereira H, Araujo RE, Barreras JV, Pangborn HC, 2021, qTSL: a Multi-Layer Control Framework for Managing Capacity, Temperature, Stress and Losses in Hybrid Balancing Systems, IEEE Trans. on Control Systems Technology, Pages: 1-16.
de Castro R, Pereira H, Araujo RE, Barreras JV, Pangborn HC, 2021, Multi-Layer Control for Hybrid Balancing Systems, IEEE Conference on Control Technology and Applications (CCTA), August 9-11, San Diego, USA (in press).
Schimpe M*, Barreras JV*, Wu B, Offer GJ, 2021, Battery Degradation-Aware Current Derating: An Effective Method to Prolong Lifetime and Ease Thermal Management, Journal of the Electrochemical Society, 168, 060506. (*Joint-first authorship)
Barreras JV, de Castro R, Wan Y, Dragicevic T, 2021, A consensus-based distributed algorithm for multi-functional battery balancing, Energies, 14, 4279.
Prosser R, Offer GJ, Patel Y, 2021, Lithium-Ion Diagnostics: The First Quantitative In-Operando Technique for Diagnosing Lithium Ion Battery Degradation Modes under Load with Realistic Thermal Boundary Conditions, Journal of the Electrochemical Society, Vol: 168, Pages: 030532
Edge JS, O’Kane S, Prosser R, Kirkaldy ND, Patel AN, Hales A, Ghosh A, Ai W, Chen J, Yang J, Li S, Pang M, Bravo Diaz L, Tomaszewska A, Marzook MW, Radhakrishnan KN, Wang H, Patel Y, Wu B, Offer GJ, 2021, Lithium Ion Battery Degradation: What you need to know, Physical Chemistry Chemical Physics.
Yu X, Jiang Y, Yang X, Cai Z, Hua Y, Yang S, Wang H, Liu X, Wang L, 2021, Dodecanethiol Coated Muti-Walled Carbon Nanotube Films as Flexible Current Collector for Lithium-Ion Batteries. Materials Letters.
Li S, Kirkaldy N, Zhang C, Gopalakrishnan K, Amietszajew T, Bravo Diaz L, Varela Barreras J, Shams M, Hua X, Patel Y, Offer GJ, Marinescu M, 2021, Optimal cell tab design and cooling strategy for cylindrical lithium-ion batteries, Journal of Power Sources, Vol 492, Pages: 229594.
Ghosh A, Foster JM, Offer G, Marinescu M, 2021, A Shrinking-Core Model for the Degradation of High-Nickel Cathodes (NMC811) in Li-Ion Batteries: Passivation Layer Growth and Oxygen Evolution, Journal of the Electrochemical Society, Vol: 168, Pages: 020509
Chowdhury R, Banerjee A, Zhao Y, Liu X, Brandon N, 2021, Simulation of Bi-layer Cathode Materials with Experimentally Validated Parameters to Improve Ion Diffusion and Discharge Capacity, Sustainable Energy Fuels, DOI: 10.1039/D0SE01611J
Gao X, Liu X, He R, Wang M, Brandon N, Wu B, Ling H, Yang S, 2021, Designed high-performance lithium-ion battery electrodes using a novel hybrid model-data driven approach, Energy Storage Materials.
The Cell Cooling Coefficient
Check out our short animation explaining this battery design tool
The cell cooling coefficient is a metric which can aid the design of the next generation of battery packs and highlight the importance of good thermal management across the battery industry.
Selected open access publications: The surface cell cooling coefficient, The tab cell cooling coefficient and How to cool Li-ion batteries