The shape of things to come
The Imperial community is reinventing the world by imagining new uses for everyday materials.
Words: Lucy Jolin
Mind-bending. World-beating. And available from a shop near you. Material scientists at Imperial are changing the look and feel of the world we will live in – on earth, in the skies and in space – with research that is designed to have an immediate impact. How are they doing it? By focusing their inventiveness on materials that are already available.
Take Elena Dieckmann (MSc Innovation Design Engineering 2016) and Ryan Robinson (PhD National Heart and Lung Institute 2016), founders of start-up Aeropowder, whose ingenious work could revolutionise the building industry using the ultimate waste product: chicken feathers.
Worldwide, we consume 134 million chickens daily: that’s 10,000 tonnes of waste feathers every day, many of which are rendered down via a high-energy process into low-grade animal feed. And yet, as Dieckmann explains, feathers are material with incredible potential. “They are made of keratin, a chemically resistant and physically strong protein. Thanks to their microscopic structure, they are one of the lightest natural fibres in nature, they are waterproof and are also excellent thermal insulators,” she says. “We realised there had to be a better use for them.”
The multi-award winning pair have developed a low-cost, low-carbon conversion process to take feathers direct from the abattoir and turn them into a range of useful products, from a water-repellent powder coating that can be applied to a range of materials to thermal insulation blocks which are not only lightweight but are biodegradable, too.
Dieckmann is currently taking her research forward in a PhD project at the Department of Civil & Environmental Engineering at Imperial, funded by the James Dyson Foundation and supervised by Professor Chris Cheeseman, Leila Sheldrick and Dr Koon-Yang Lee. Her co-founder Ryan Robinson is working full-time exploring the company’s commercial potential.
But while the applications are immediately obvious, Dieckmann says the process of actually getting an idea to market has been eye-opening. “What happens in industrial testing and what happens in the lab can be two very different things!”
Dr Koon-Yang Lee, Senior Lecturer in the Faculty of Engineering, says that the Aeropowder approach has huge implications for material sciences. “What we’re doing is taking whatever is available right now. We try to perform basic modifications or create simpler solutions which lead to the same outcome – better performing materials,” he says.
And that has real, immediate benefits for researchers. First, it’s easier to modify something that already exists rather than create something entirely new. Second, it’s a quicker way to commercialise a product.
Lee points out that the compound polytetrafluoroethylene, which forms the basis of Teflon, was discovered by chemical company DuPont employee Roy Plunkett in 1938, but the first Teflon-coated ‘Happy Pan’ didn’t go on sale until 1961.
“If you take existing materials, this allows you to solve problems in the immediate future, rather than waiting for the years of basic R&D needed when developing new solutions,” he says.
Dr Ben Britton, Senior Lecturer in the Department of Materials, studies materials at the atomic level – how they bond together and how their structure performs. But his focus is on what the atomic scale means for life at the human one. “Changes at the smallest scale can have a huge impact on structures where failure could be catastrophic: aerospace jet engines, oil and gas pipelines and nuclear reactors,” he says.
We try to perform basic modifications or create simpler solutions which lead to the same outcome – better performing materials"
Dr Koon-Yang Lee
One of the most important aspects of running a nuclear reactor, for example, is making sure all the bits and pieces stay in one piece, especially with the UK’s ageing reactor fleet, he explains. The reactors need to run for as long as possible, to maximise their life. “We need to predict when the materials will fail, so that we can rotate the parts out of service and put fresh parts in. In a jet engine, we look at the integrity of the disc and fan blades at the very front of an engine. And we look at pipelines and cutting materials in oil and gas – when you are drilling you want to make sure you can maximise the amount of time spent drilling. My colleague Dr Finn Giuliani and I are trying to understand the failure mechanisms in tools that sit on the front of the drill and bore into the rock.”
Much of this work involves studying samples of materials that have failed or are likely to fail, and examining exactly what happens to them under the microscope when they’re subjected to stress. “If you’ve ever been bored and bent a spoon back over and over again, you’ll know that the spoon will eventually break,” says Britton. “But in fact, that’s not because you applied so much deformation. It’s that you’ve effectively exhausted the plasticity within that material. Each time you fly a plane – taking off, going into cruise and then landing – you’re bending the spoon.”
Indeed, materials that go into orbit have to be able to cope with hugely demanding environments, both while they’re out in space and when they’re sent through the Earth’s atmosphere. Understanding exactly how well they cope – and when they stop coping – is essential. Which is why Britton and Giuliani have recently developed a novel project to test exactly how well silicon carbide – a light, stable ceramic used in space telescope mirrors – performs under stress.
“We cut a square cross-section pillar-shaped object of silicon carbide, two micrometres by two micrometres by six micrometres,” Britton explains. “Then we introduce a tiny crack in the top of that pillar, and push a diamond wedge into that crack. We watch in real time using the electron microscope. We measure the energy that dissipates as that crack progresses. This means we can understand the toughness: how high we can drop something before it cracks and how much weight we can put on an object before it breaks – essential knowledge when you’re flying a satellite into space.”
Feathers and silicon carbide both occur in nature – but synthetic materials are also ripe for improvement and reinvention. Foams, for example, are used widely where weight saving is critical, such as in aeroplanes. Currently, high-performance foams are produced in a block and shaved down into the required shape, while low-performance foams, such as those used in everyday packaging, are injection moulded into the desired shape. Lee’s team have created a foam that combines the properties of both high and low – a foam that is strong enough to withstand the stress of an aerospace application but can be moulded to any exact shape, thus reducing cost and waste shavings.
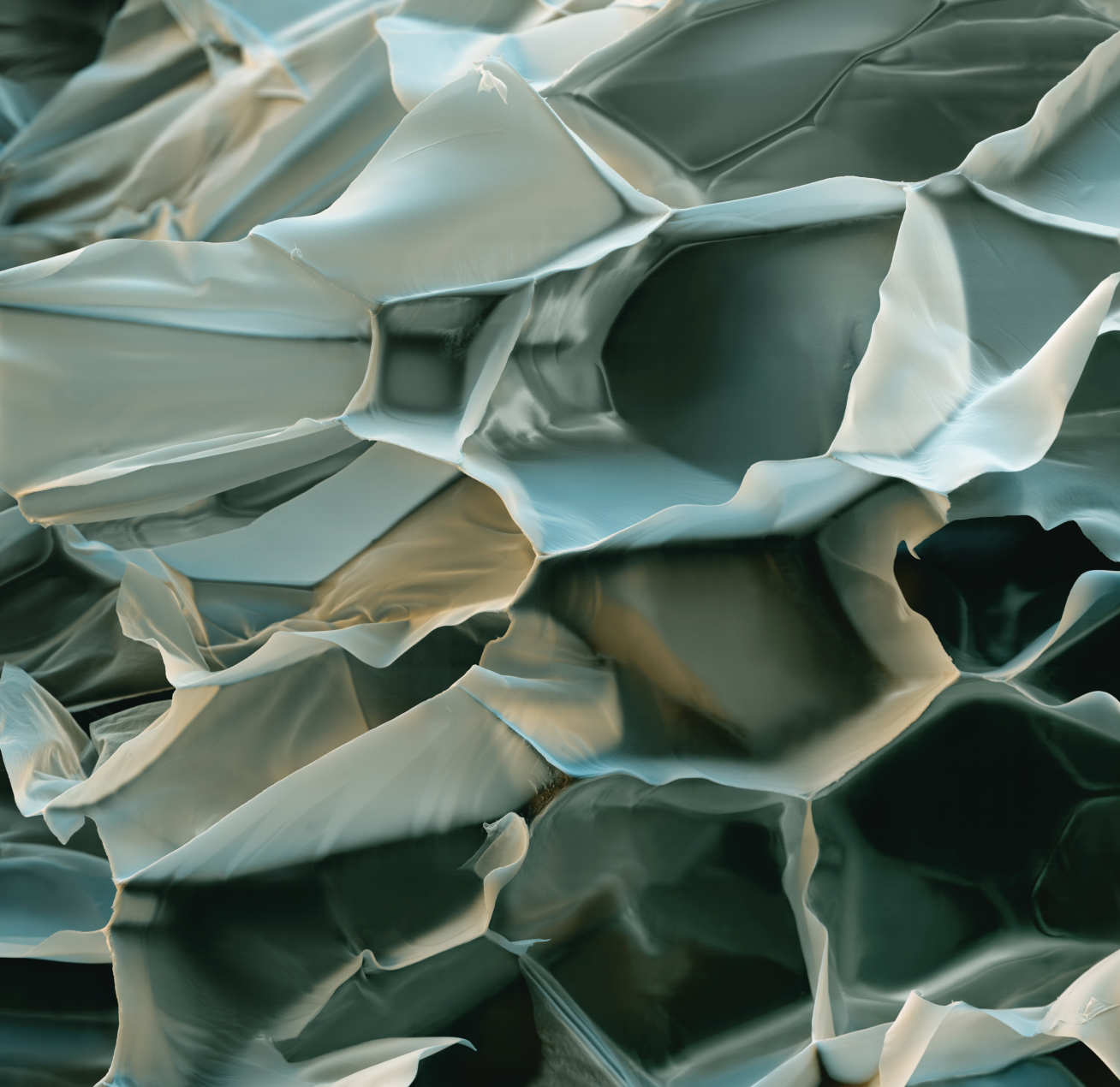
If you’ve ever made a meringue, you’ll be familiar with the process: it begins life as a liquid. Air is beaten into it so that it becomes thicker and easier to shape. “You can shape it into anything, from a gingerbread man shape to an aerofoil shape,” Lee explains. “That automatically leads to cost saving, as you are not going to make a block and then shave it into the shape of a gingerbread man. You can just pull it directly into the gingerbread man mould. And in most processes, you have to modify your starting liquid to create a foam by adding, for example, five per cent of a blowing agent – a chemical that makes bubbles in a polymer foam. With ours, all we have to do is beat it, shape it and let it set. You don’t need anything else other than a whisk and a mould.”
Much of this work is done in close collaboration with industry, seeking real-world solutions for real-world problems. But that’s not the only partnership needed: this kind of innovation also reaches across many other disciplines: chemistry, physics, engineering and materials sciences.
“It’s really nice to sit on that cusp between science and engineering, where we can take engineering problems, apply scientific approaches and try to deliver something where we have a new understanding that companies and collaborators can gain from,” says Britton. “We all like flying. We all like electricity. We all want our materials to perform better.”