When Imperial researchers turned decades of research into the way our lungs function on its head, the medical world refused to believe. This is what happened next.
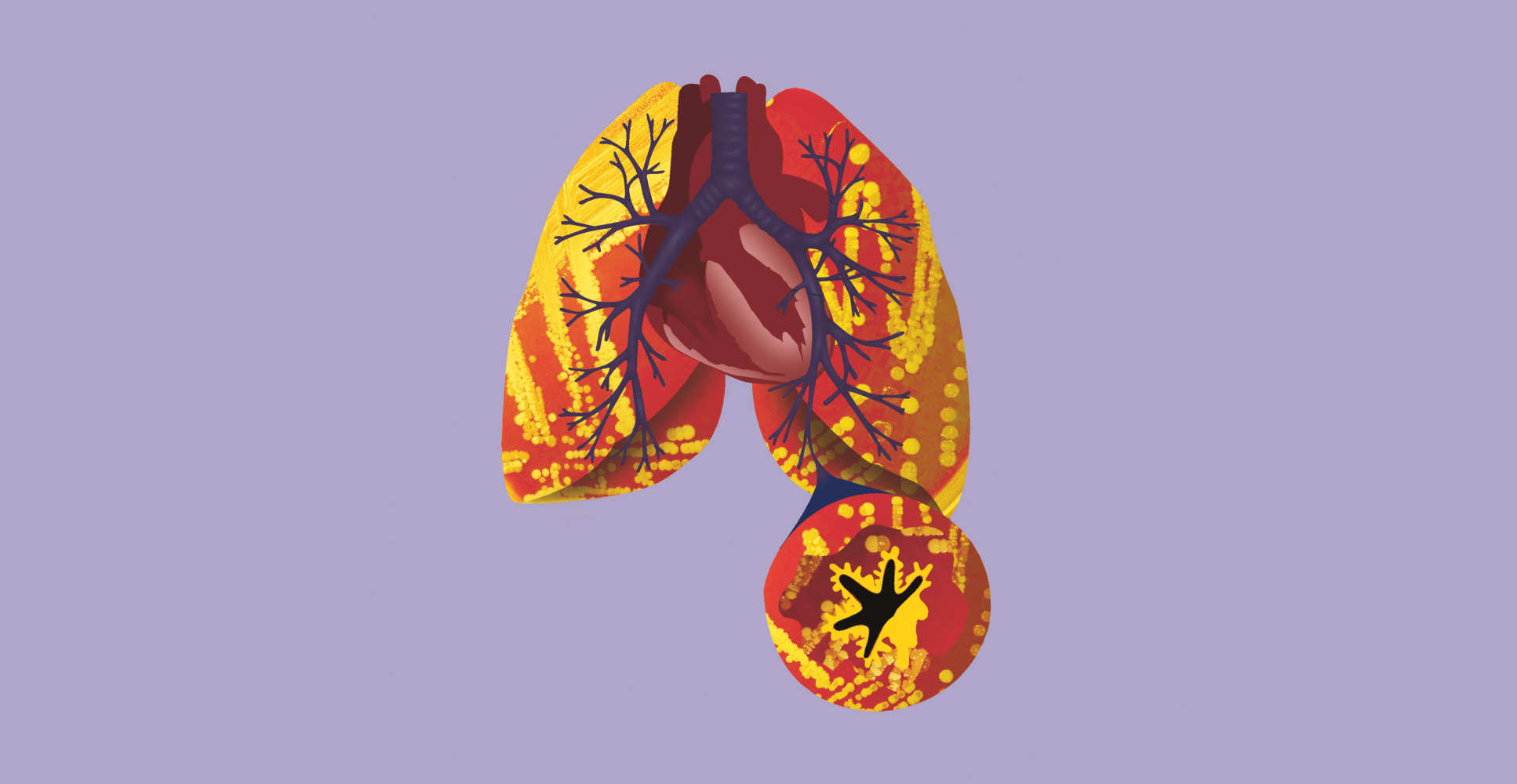
Words: Becky Allen / Illustrations: Sarah Wickings
In 2010, a pair of Imperial professors rewrote the medical textbooks. Their paper, analysing the characteristics of asthma patients, overturned everything we know – or thought we knew – about how our lungs function.
Indeed, what Miriam Moffatt, Professor of Respiratory Genetics, and William Cookson, Professor of Genomic Medicine, discovered was so unexpected that a string of scientific journals rejected their paper.
“It took us two years to get our paper published because lots of journals said the lungs are sterile – and that our results were nonsense,” Cookson remembers. We’ve grown accustomed to the idea that we need to care for the hundreds of other species that share our guts, but when it came to the airways and lungs, there was an assumption that it was a sterile environment – in other words, devoid of all microbes. “But we found lots of bugs down there. In healthy people’s lungs we found a characteristic community of microbes, and in asthma we found a very different microbiota.”
Researchers have long recognised that a rich microbial environment protects against asthma, but Moffatt and Cookson wondered whether it might be down to the microbes in the lungs modifying our immune system. So, together with colleagues from Imperial and Dublin’s Connolly Hospital, they set out to sample the lung microbiome in people with and without asthma.
With hindsight, the idea of sterile lungs seems pretty far-fetched. Even at rest, we inhale 10,000 litres of air a day, and air is far from sterile. In cities, every litre of it contains up to 100,000 bacteria. Yet, in 2008, when the Human Microbiome Project began mapping the microbial communities of hundreds of healthy humans, it omitted every part of the respiratory tract – from the nose to the nether reaches of the lung.
There are good reasons the textbooks were so wrong, for so long. First, lungs are inaccessible places. Whereas gut researchers have a ready supply of raw material to work on, collecting samples from the airway involves a hospital visit for a bronchoscopy. Second, until the relatively recent advent of affordable DNA-sequencing technology, researchers relied on 19th-century culture methods, tools that detect only a small subsection of the microbes in lung samples. Despite the amount of air we breathe, there are parts of the lung that get little oxygen and, here, anaerobic organisms thrive unseen.
According to Moffatt: “They are not oxygen lovers, so they need very special anaerobic culture that’s not typically done in the microbiology lab. That explains why they’d not been seen before – because only five per cent can be cultured using standard techniques.”
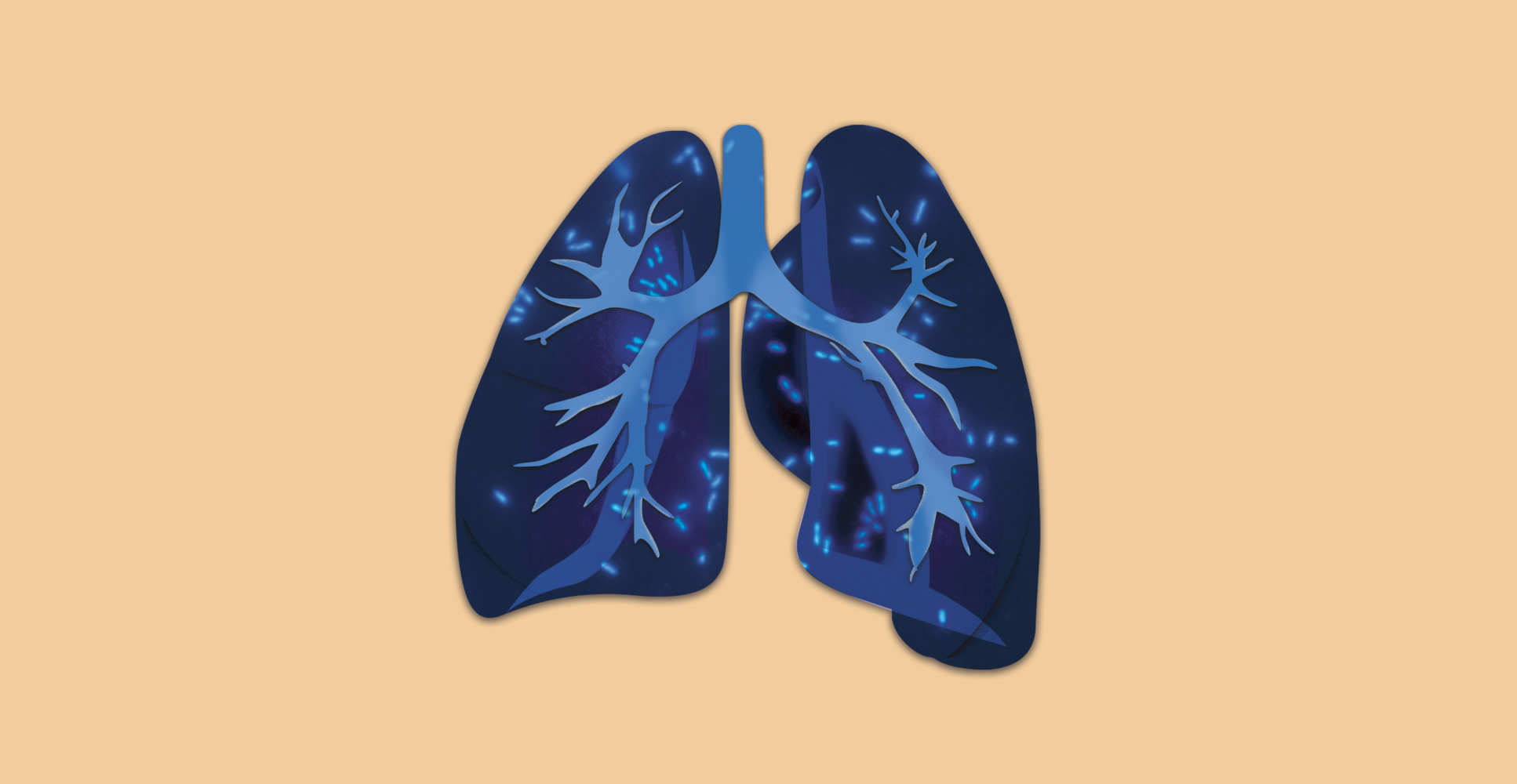
Discovering that we share our lungs with all these other organisms poses a whole new set of questions – and opportunities – for lung researchers: what are all these microbes doing down there? How do their communities differ between health and disease? And how might this knowledge lead to new ways of diagnosing and treating lung disease?
Making progress matters because lung disease is a huge global problem. Asthma affects 300 million people worldwide and chronic obstructive pulmonary disease (COPD), 64 million. Respiratory infections are the leading cause of death in developing countries, killing more than four million people a year. Added to this, antibiotics used to treat lung infections are fuelling the global crisis of antibiotic resistance.
Similar microbiome changes have already been found in a range of lung diseases. Jane Davies, Professor in Paediatric Respirology and Experimental Medicine at Imperial’s National Heart and Lung Institute (NHLI), has found it in cystic fibrosis (CF). The nature of the disease means Davies is familiar with seeing microbes in her patients’ lungs. In CF, a genetic mutation causes the production of large amounts of mucus, trapping bacteria and viruses in the lungs and resulting in inflammation and irreversible lung damage.
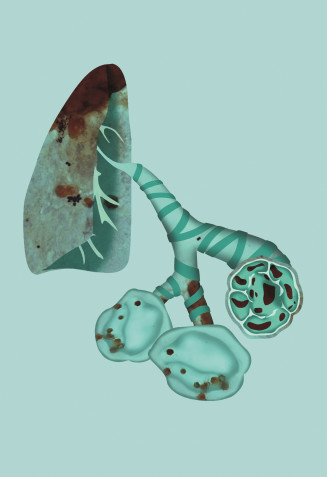
Using new molecular tools to study the lung microbiome has changed her view of CF. “Patients start with a mixed, diverse community of microorganisms and, over 20 years, this healthy diversity decreases in line with their evolving lung disease. The more severe the disease, the lower the diversity,” she says. “What’s new for us – but not to gut researchers – is that in any ecological niche, diversity is good because it helps keep things in balance. Once it becomes disrupted, certain organisms can become dominant and cause trouble.”
For Davies, the challenge now is to understand these changes so that they can be used to improve treatment in CF: “What’s most important for me as a clinician is what these findings mean. We still don’t know. So far it’s not changed how we treat patients and isn’t helping us with prognosis – these are the burning issues.”
The challenge for respiratory professor Toby Maher, British Lung Foundation Chair in Respiratory Research at Imperial, focuses on a disease of older age: idiopathic pulmonary fibrosis (IPF). Partly hereditary – about 50 per cent is genetic – it is also caused by a lifetime of physical damage to the lung, such as working in dusty conditions. The lungs become progressively scarred, and, as they shrink and stiffen, patients becoming more and more breathless.
The puzzle for Maher is IPF’s hugely variable progression. Some patients deteriorate rapidly, while in others the disease is more stable. “I want to understand the factors that contribute to IPF’s development and progress, so that ultimately we can intervene and treat patients,” he explains. “Studying the microbiome has allowed us to explore environmental factors that may be responsible for some of the differences we see between patients.”
Maher is also studying how the immune system contributes to fibrosis. “There’s clearly an important overlap between the microbiome and the host immune system. Research in the gut tells us it’s a two-way process, he says, “so we’re trying to understand how the relationship between the immune system and colonising bacteria affects disease progression”.
COPD is another lottery of a lung disease, progressing very rapidly in some patients and more slowly in others. COPD kills around 30,000 people a year in the UK, and is the third-highest cause of death worldwide.
Largely smoking-related, it is one of the commonest causes of medical hospital admission, and is associated with many other conditions, from heart disease and diabetes to osteoporosis and cognitive decline. “COPD is a complex disease in which the microbiome seems to play a crucial role,” says Wisia Wedzicha, Professor of Respiratory Medicine at Imperial’s NHLI. “And it is a very important condition because it often affects people who are still working. Patients become breathless – which is a horrible symptom – and our interventions are very limited at the moment. We really have nothing that affects disease progression.”
For the past 20 years, Wedzicha has been studying sudden flare-ups in COPD, because these exacerbations cause the disease to progress more rapidly. “Exacerbations are caused by infections such as cold and flu,” she says, “and we’re only now discovering these exacerbations are also associated with changes in the microbiome, which could help lead to earlier diagnosis and better treatment.”
We still have more to discover about what the lung microbiome does, but, from what we know about the gut, these bugs probably work in tandem with human cells to form a healthy immune system. If that turns out to be correct, Moffatt and Cookson believe that it will change how we diagnose and treat lung disease, and help us tackle antibiotic resistance.
“In two to three years we’re going to have a range of diagnostic tools that can quickly diagnose lung infections, not just in asthma but in children with CF who have a complicated mix of pathogens,” says Moffatt. “Treatment regimes have been based on the idea that lungs are sterile and you get a single pathogen, but actually they have a soup of pathogens, so DNA sequencing will change clinical management of acute and chronic infections.”
Quick and accurate diagnosis should also cut unnecessary antibiotic use, as prescribing will become more precisely tailored to the pathogens that are actually present. Moffatt and Cookson also plan to develop inhaled antibiotics that do not damage the gut microflora and will, therefore, help prevent antimicrobial resistance.
In the longer term, their rewriting of the textbooks could herald revolutionary new ways of treating lung disease, including phages (viruses that attack bacteria) and – most speculative of all – transplanting healthy microbial communities into diseased airways. “I don’t know when,” Cookson concludes, “but I’m sure it will happen, and sooner rather than later.”